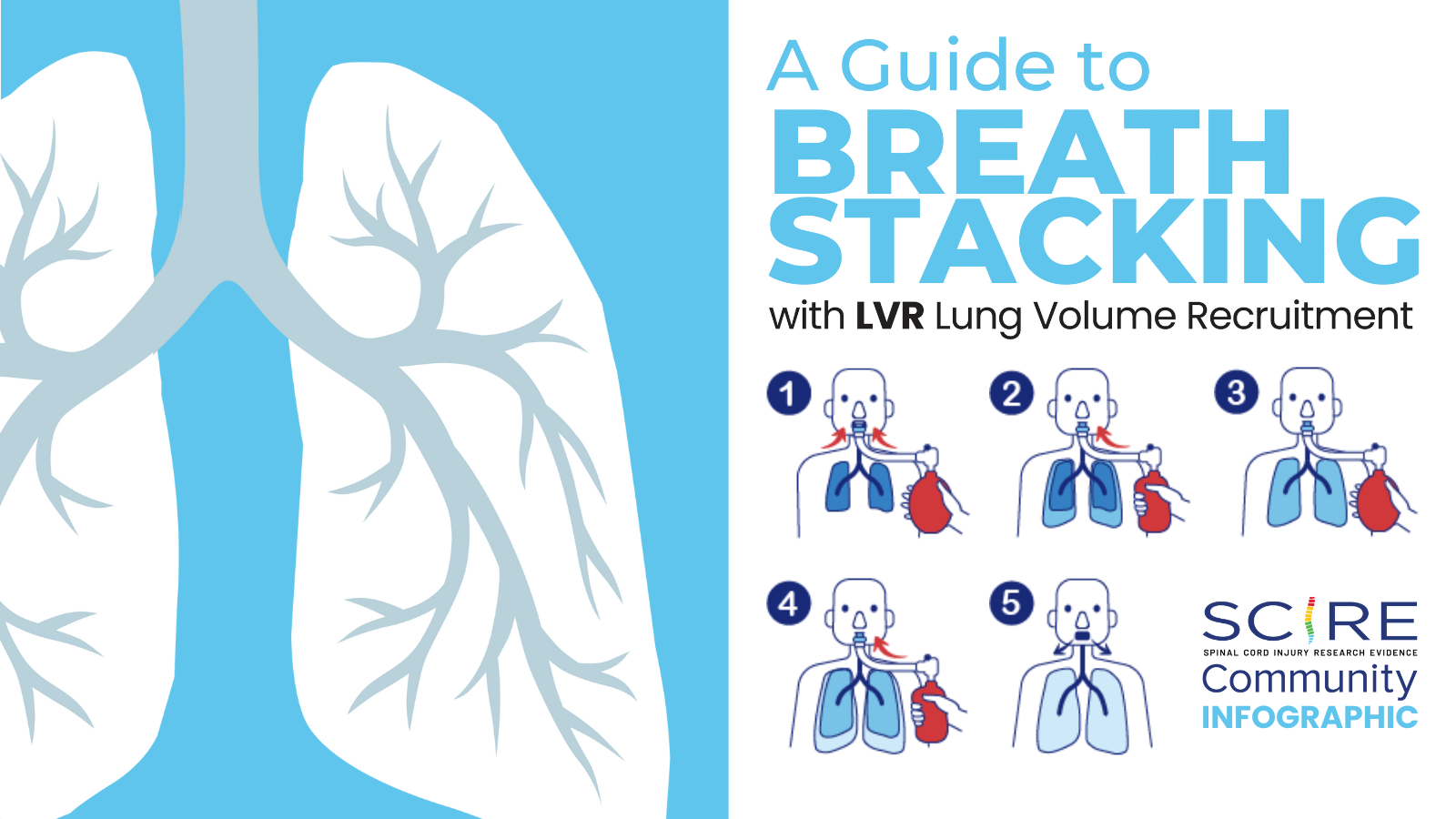
Author: Kelsey Zhao | Reviewers: GF Strong OTs and PTs | Published: 30 January 2025 | Updated: ~
Author: Kelsey Zhao | Reviewers: GF Strong OTs and PTs | Published: 30 January 2025 | Updated: ~
Author: Kelsey Zhao | Reviewers: GF Strong OTs and PTs | Published: 11 June 2024 | Updated: ~
For more information on abdominal binders, visit: community.scireproject.com/topic/abdominal-binders/
Authors: Dominik Zbogar and Sharon Jang | Reviewer: Susan Harkema | Published: 14 February 2022 | Updated: ~
Key Points
Neuromodulation is a general term for any treatment that changes or improves nerve pathways. Different types of neuromodulation can work at different sites along the nervous system (e.g., brain, nerves, spinal cord) and may or may not be invasive (i.e., involve surgery). Epidural stimulation (also known as epidural spinal cord stimulation or direct spinal cord stimulation) is a type of invasive neuromodulation that stimulates the spinal cord using electrical currents. This is done by placing an electrode on the dura (the protective covering around the spinal cord).
To read more about other types of neuromodulation used in SCI, access these SCIRE Community articles: Functional Electrical Stimulation (FES), Transcutaneous Electrical Nerve Stimulation (TENS), sacral nerve stimulation, and intrathecal Baclofen.
![]() | ![]() |
![]() | ![]() |
Watch our neuromodulation series videos! Our experts explainexperimental to more commonplace applications, and individuals with SCI describe how neuromodulation has affected their lives.
Epi- is a prefix and means “upon”, and the dura (full name: dura mater) is a protective covering of the spinal cord. So epidural means “upon the dura”, and in the context of epidural stimulation, this is where the electrodes that stimulate the spinal cord are placed. Yes, it is also possible to have sub-dural (under the dura) or endo-dural (within the dura) electrode placement. And, there are more layers between the dura and the spinal cord, not to mention the spinal cord itself where electrodes could be placed in what is called intraspinal microstimulation. The benefit of being beneath the dura and closer to the spinal cord is that there is a more direct stimulation. Having the electrode closer to the spinal cord allows more precision with the signal going more directly to the neurons.
The drawback is that more complications can arise with closer placement because the electrodes are in the spinal cord tissue. Such placement is currently rare, experimental, or non-existent but that will change as the technology advances. Intraspinal microstimulation has been tested in animal models and is in the process of being translated to humans.
You are probably familiar with the term “epidural” already, as it is often mentioned in relation to childbirth. If a new mother says she had an epidural, what she usually means is that she had pain medication injected into the epidural space for the purpose of managing pain during birth.
We specifically discuss epidural spinal cord stimulation in this article. Spinal cord stimulation can also be applied transcutaneously. This type of spinal cord stimulation is non-invasive as the stimulating electrodes are placed on the skin. With transcutaneous stimulation, the signal has to travel a greater distance through muscle, fat, and other tissues, which means the ability to be precise with stimulation is hampered. However, it does allow for more flexibility in electrode placement and does not require surgery. There is research published or underway investigating the impact of transcutaneous stimulation in some of the areas discussed above, including hand, leg, and cardiovascular function.
Normally, input from your senses travels in the form of electrical signals through the nerves, up the spinal cord, and reaches the brain. The brain then tells the muscles or organs what to do by sending electrical signals back down the spinal cord. After a spinal cord injury, this pathway is disrupted, preventing electrical signals from traveling below the level of injury to reach where they need to go. However, the nerves, muscles, and organs can still respond below the injury to electrical signals.
Epidural stimulation works by helping the network of nerves in the spinal cord below the injury function better and take advantage of any leftover signals from the spinal cord. To do so, the stimulation must be fine-tuned to make sure the amount of stimulation is optimal for each person and a specific function, such as moving the legs.
Recent studies of the role of epidural stimulation on standing and walking have noted unexpected beneficial changes in some participants’ bowel, bladder, sexual, and temperature regulation function. This highlights both the potential for epidural stimulation to improve quality of life in multiple ways and that much research remains to be done to understand how epidural stimulation affects the body.
There may still be spared connections in the spinal cord with a complete injury.
Being assessed with a complete injury implies that there is no spared function below the injury. However, scientists are finding that this may not be the case. Studies have found that even with a complete loss of sensory and motor function, there may be some inactive connections that are still intact across the injury site. These remaining pathways may be important for regaining movement or other functions. Another hypothesis is that epidural stimulation in combination with training may encourage stronger connections across the level of injury. Although these pathways may provide some substitution for the injured ones, they are not as effective as non-injured pathways across the injury level.
When it is decided that an individual will receive epidural stimulation, a health professional, such as a neurosurgeon, will perform an assessment of the spinal cord using magnetic resonance imaging (MRI) to determine the best place to implant the electrodes.
In most of the studies mentioned in this article, the electrodes were placed between the T9-L1 levels, though researchers are investigating the impact of epidural stimulation on hand function.
Xray image of wires connecting power and signal to electrodes (red circle) placed on a spinal cord.
There are two possible procedures. One approach is to have two surgeries. During the initial surgery, a hollow needle is inserted through the skin into the epidural space, guided using fluoroscopy, a type of X-ray that allows the surgeon to see where the needle is in real time. Potential spots on the spinal cord are tested using a stimulator. A clinician will look to see if stimulation over those areas of the spinal cord leads to a desired response. Once found, the electrode array is properly positioned over the dura and the surgery is completed. This begins a trial period where the response to epidural stimulation is monitored. During this time the electrode array is attached to an electrical generator and power supply, which is worn on a belt outside of the body. When it is shown that things are working as desired, the generator is implanted underneath the skin in the abdomen or buttocks. The generator can be rechargeable or non-rechargeable. A remote control allows one to turn the generator on or off and control the frequency and intensity of stimulation.
The second method is to only have one surgery and no trial period. This is possible due to increased knowledge in how to stimulate the spinal cord. Soon after surgery, the individual will be taught how and when to use the epidural stimulation system at home. If needed, the frequency (how often) and intensity (how strong) of the stimulation will be adjusted at follow-up appointments with the physician. In other cases, many practice sessions of learning the right way to stimulate may be needed before a person can stimulate at home.
If the epidural stimulation is used for leg control, movement training, standing, and stepping will be required to learn how to coordinate and control movement during stimulation. This is required for the recovery of voluntary movements, standing and/or walking.
Epidural stimulation can be used in all people with SCI, regardless of the level or completeness of injury. However, certain situations can make it an unsafe treatment in some. It is important to speak to a health professional about your health history before beginning any new treatment.
Epidural stimulation is generally well-tolerated, but there is a risk of experiencing negative effects.
Proper training on how to use the equipment and using the stimulation according to the directions of your health provider can help decrease the risks of experiencing these side effects.
Neuromodulation methods to manage bladder function have usually involved stimulation of the sacral nerves (which are outside of the spinal cord), not with epidural spinal cord stimulation. This is reflected in the fact that almost no research exists regarding the effects of epidural stimulation on bowel and bladder function in the previous century.
New information on epidural stimulation relating to bladder function is coming. In the last several years, several studies (weak evidence) from a very small group of participants of participants (who were AIS A or B) have found consistent improvements in bladder function. Participants in these reports were fitted with epidural stimulators for reactivation of paralyzed leg muscles for walking and reported additional benefits of improvements in bladder and/or bowel function. However, other studies have shown small changes to bladder function and no changes to bowel function. Negative changes, such as decreased control over the bladder, have even been noticed by some participants in another study. These findings suggest that epidural stimulation may improve quality of life by safely increasing the required time between catheterizations. Fewer catheterizations and reduced pressure in the bladder would preserve lower and upper urinary tract health. More research is required, especially with respect to bowel function. It must be noted that walk training alone has been shown to improve bladder and bowel function. Epidural stimulation may provide additional improvement to bladder function in comparison to walk training alone. Neuromodulation methods to manage bladder function have usually involved stimulation of the sacral nerves (which are outside of the spinal cord), not with epidural spinal cord stimulation. This is reflected in the fact that almost no research exists regarding the effects of epidural stimulation on bowel and bladder function in the previous century.
For more information, visit our pages on Bowel and Bladder Changes After SCI!
Relationships between the leg movement and nerves in the low back regions have been identified.
Some evidence suggests that walk/step training alone can create improvements in bladder/bowel function. Researchers hypothesize that the sensory information created through walking or standing provides stimulation to the nerves in the low back region, which contains the nerves to stimulate bowel, bladder, and sexual function. Research has shown that bending and straightening the legs can be enhanced by how full the bladder is and the voiding of urine.
One of the consequences of SCI is the loss of muscle mass below the injury and a tendency to accumulate fat inside the abdomen (abdominal fat or visceral fat) and under the skin (subcutaneous fat). These changes and lower physical activity after SCI increase the risk for several diseases.
A single (weak-evidence) study measured body composition in four young males with complete injuries. Participants underwent 80 sessions of stand and step training without epidural stimulation, followed by another 160 sessions of stand/step training with epidural stimulation. This involved one hour of standing and one hour of stepping five days a week. After all, training was complete, all four participants had a small reduction in their body fat, and all participants but one experienced an increase in their fat free body mass (i.e., the weight of their bones, muscles, organs, and water in the body) in comparison to their initial values prior to stimulation. While all participants experienced a reduction of fat, the amount of fat loss was minimal, ranging from 0.8 to 2.4 kg over a period of a year.
The first use of epidural stimulation was as a treatment for chronic pain in the 1960s. Since then, it has been widely used for chronic pain management in persons without SCI. However, it is important to recognize that the chronic pain experienced by those without SCI is different from the chronic neuropathic pain experienced after SCI. This may explain, to some extent, why epidural stimulation has not been as successful in pain treatment for SCI. The mechanism by which electrical stimulation of the spinal cord can help with pain relief is unclear. Some research suggests that special nerve cells that block pain signals to the brain may be activated by epidural stimulation.
There are a few studies focused on the role of epidural stimulation in managing pain after SCI. A number of other studies included a mix of different people with and without SCI. Because chronic neuropathic pain after SCI may not be the same as the chronic pain others experience, studies that do not separate mixed groups raise questions about the validity of findings. The number of individuals with SCI in these studies is often small, most were published in the 1980s and 1990s and so are quite dated, and the research is classified as weak evidence.
The results of this body of research show that some people may receive some pain reduction. Those who saw the most reduction in pain were individuals with an incomplete SCI. Also, satisfaction with pain reduction drops off over time. One study showed only 18% were satisfied 3 years after implantation. A different study looking at the long-term use of epidural stimulation for pain reduction found seven of nine individuals stopped using this method.
In the only recent study in this area, one woman with complete paraplegia (weak evidence) experienced a reduction in neuropathic pain frequency and intensity, and a reduction in average pain from 7 to 4 out of 10, with 0 being no pain and 10 being the worst imaginable pain. This improvement remained up to three months later after implantation of the epidural stimulation device.
It should be noted that the studies for pain place electrodes in different parts of the spinal cord compared to the more recent studies for voluntary movement, standing and stepping.
Refer to our article on Pain After SCI for more information!
Using epidural stimulation to improve respiratory function is useful because it contracts the diaphragm and other muscles that help with breathing. Also, these muscles are stimulated in a way that imitates a natural pattern of breathing, reducing muscle fatigue. More common methods of improving respiratory function do not use epidural stimulation, but rather, directly stimulate the nerves that innervate the respiratory muscles. While such methods significantly improve quality of life and function in numerous ways, they are not without issues, including muscle fatigue from directly stimulating the nerves.
To date, most research into using epidural stimulation to improve respiratory function has been done in animals. Recently, research has been done in humans and weak evidence suggests that epidural stimulation may:
Long term use of epidural stimulation shows that improvements remain over years and that minimal supervision is needed, making it suitable for use in the community.
Refer to our article on Respiratory Changes After SCI for more information!
The impact of epidural stimulation on sexual function has been a secondary focus in research studies looking at standing and walking. Currently, there are reports from one male and two females.
After a training program of walk training with epidural stimulation, one young adult male reported stronger, more frequent erections and the ability to reach full orgasm occasionally, which was not possible before epidural stimulation. However, this study looked at the effects of walk training and epidural stimulation together, which took place after several months of walk training without stimulation. Because the researchers did not describe what the individual’s sexual function was like after walk training, it is difficult to say how much benefit is attributed to epidural stimulation versus walk training.
In another study with two middle-aged females 5-10 years post-injury, one reported no change in sexual function and the other reported the ability to experience orgasms with epidural stimulation, which was not possible since her injury.
Refer to our article on Sexual Health After SCI for more information!
Botulinum toxin (Botox) injections and surgically implanted intrathecal Baclofen pumps are the most common ways to manage spasticity. Baclofen pumps are not without issues, however. Many individuals do not qualify for this treatment if they have seizures or blood pressure instability, and pumps require regular refilling.
Research in the 80s and 90s on the use of epidural stimulation for spasticity did not report very positive findings. It was noted that greater benefits were found in those with incomplete injury compared to those who were complete. Another paper concluded that (weak evidence) the beneficial effects of epidural stimulation on spasticity may subside for most users over a short period of time. This, combined with the potential for equipment failure and adverse events, suggested that epidural stimulation was not a feasible approach for ongoing management of spasticity.
More recently, positive results with epidural stimulation have been observed (weak evidence). This is likely due to improvements in technology, electrode placement, and stimulation parameters. Positive findings show that participants:
For more information, visit our page on Botulinum Toxin and Spasticity!
In a study with a single participant (weak evidence) investigating walking, an individual implanted with an epidural stimulator also reported improvement in body temperature control, however details were not provided. More research is required to understand the role of epidural stimulation for temperature regulation.
In severe SCI, individuals may suffer from chronic low blood pressure and orthostatic hypotension (fall in blood pressure when moving to more upright postures). These conditions can have significant effects on health and quality of life. Some recent studies have looked at how epidural stimulation affects cardiovascular function to improve orthostatic hypotension. Overall, they show (weak evidence) that epidural stimulation immediately increases blood pressure in individuals with low blood pressure while not affecting those who have normal blood pressure. They also showed that there is a training effect with repeated stimulation. This means that after consistently using stimulation for a while, normal blood pressure can occur even without stimulation when moving from lying to sitting.
Moreover, researchers are starting to believe that changes in orthostatic hypotension and blood pressure can promote changes in the immune system (Bloom et al., 2020). In the body, the blood helps to circulate immune cells so they are able to fight infections in various areas. One case study found that after 97 sessions of epidural stimulation, the participant had fewer precursors for inflammation and more precursors for immune responses. Although these changes are exciting, researchers are still unsure why this happens, and whether these effects occur with all people who are implanted with an epidural stimulator.
Refer to our article on Orthostatic Hypotension for more information!
For individuals with tetraplegia, even some recovery of hand function can mean a big improvement in quality of life. Research into using epidural stimulation to improve hand function consists of one case study (weak evidence) involving two young adult males who sustained motor complete cervical spinal cord injury over 18 months prior.
The researchers reported improvements in voluntary movement and hand function with training while using epidural stimulation implanted in the neck. Training involved grasping and moving a handgrip while receiving stimulation. For 2 months, one man engaged in weekly sessions while the other trained daily for seven days. One participant was tested for a longer time as a permanent electrode was implanted, while the other participant only received a temporary implant. Both participants increased hand strength over the course of one session. Additional sessions brought additional gradual improvements in hand strength as well as hand control (i.e., the ability to move the hand precisely). These improvements carried over to everyday activities, such as feeding, bathing, dressing, grooming, transferring in and out of bed and moving in bed. Notably, these improvements were maintained when participants were not using epidural stimulation.
Being able to control your trunk (or torso) is important for performing everyday activities such as picking things up or reaching for items. One study found that using epidural stimulation can increase the amount of distance you are able to lean forward. The improvement in forward reach occurred immediately when the stimulation was turned on. The two participants in this study were also able to reach more side to side as well, but the improvement was minor.
Voluntary movements (i.e., being able to move your body when you want to) of affected limbs can occur with the use of epidural stimulation. Researchers are still unsure of the right training regimen to optimize results. For example, one study found that many sessions of step training with epidural stimulation are required for participants to slowly regain voluntary movement of the leg and foot with epidural stimulation when lying down. However, another study found that participants were able to voluntarily move their legs with stimulation and no stand training.
Voluntary movements (i.e., being able to move your body when you want to) of affected limbs can occur with the use of epidural stimulation. Researchers are still unsure of the right training regimen to optimize results. For example, one study found that many sessions of step and stand training with epidural stimulation are required for participants to slowly regain voluntary movement of the leg and foot with epidural stimulation when lying down. However, another study found that participants were able to voluntarily move their legs with stimulation and no stand training though the amount each participant was able to move their legs with epidural stimulation varied greatly. For example, one participant was able to voluntarily move their leg without any stimulation after over 500 hours of stand training with epidural stimulation while another participant from the same study was not able to voluntarily move their leg without stimulation after training. Overall, more than 25 people can move some or all of their leg joints voluntarily from the first time they receive epidural stimulation.
More recently, research shows that some with epidural stimulators can produce voluntary movements without stimulation on and without any intensive training program. In one study, participants did not do a consistent intensive training program, although many of them attended out-patient therapy or did therapy at home. Over the period of a year, 3 of 7 participants were able to voluntarily bend their knee, and bend and straighten their hips. Additionally, of those 3 participants, 2 were able to point their toes up and down. While the number of people able to make voluntary movements without stimulation is small, many more studies are underway.
Recent research indicates that epidural stimulation can influence walking function in individuals with limited or no motor function. While these findings are exciting, researchers are still learning how to use stimulation effectively to produce walking motions. Before being able to walk again, people must be able to make voluntary movements and be able to stand.
Some studies have also found that with extensive practice (e.g., 80 sessions), independent standing (i.e., standing without the help of another person, but holding onto a bar) may be achieved without epidural stimulation. Gaining the ability to stand may also occur with stand training combined with epidural stimulation. However, the findings with regard to the effect of stand training with epidural stimulation have been mixed. For example, one study showed that stand training for 5 days a week over a 4 month period with epidural stimulation resulted in independent standing for up to 10 minutes in an individual with a complete C7 injury, while another study has suggested that independent standing for 1.5 minutes can be achieved with epidural stimulation and 2 weeks of non-step specific training in an individual with complete T6 injury.
Earlier research has found that epidural stimulation can help with the development of walking-like movements, but these movements do not resemble “normal” walking. Instead, they resemble slight up and down movements of the leg. Recent studies have shown that with 10 months of practicing activities while lying down on the back and on the side, in addition to standing and stepping training, people are able to take a step without assistance from another person or body weight support. While some individuals in these studies have been able to regain some walking function, they are walking at a very slow pace, ranging from 0.19 meters per second to 0.22 meters per second. This is much slower than the 0.66 meters per second required for community walking. For example, of the 4 participants in one study, two were able to walk on the ground with a walker, one was only able to walk on a treadmill, and one was able to walk on the ground while holding the hands of another person. These differences in walking abilities gained by participants were not expected.
In late 2018, one researcher demonstrated that constant epidural stimulation was interfering with proprioception, or the body’s ability to know where your limbs are in space, which ultimately hinders the walking relearning process. The solution to this problem involves activating the stimulation in a specific sequence, rather than having it continuously on. With this method and a year’s worth of training, participants were able to begin walking with an assistive device (such as a walker or poles) without stimulation. However, these individuals had to intensively practice standing and walking with stimulation for many months to produce these results. In these studies, one case of injury was reported where a participant sustained a hip fracture during walking with a body weight support. Further studies on how to individualize therapy will be necessary as the response to treatment in these studies varied greatly from person to person depending on the frequency and intensity of the stimulation.
Most of the stand/walk training conducted in the studies is with the use of a body weight support treadmill.Is it the training or the epidural stimulation?
Arm and leg movement and blood pressure have been seen to improve with epidural stimulation, but the role of rehabilitation in these recoveries is unclear. Rehabilitation techniques can have an effect on regaining motor function. For example, step/walk training alone can help improve the ability to make voluntary movements, walking and blood pressure among individuals with incomplete injuries. In much of the current research, epidural stimulation is paired with extensive training (typically around 80 sessions) before and after the epidural stimulator is implanted. Furthermore, these studies do not compare the effects of epidural stimulation to a control group who receives a fake stimulation (a placebo) which would help to see if stimulation truly has an effect. Without this comparison, we are unable to clearly understand the extent of recovery that is attributable to epidural stimulation versus the effects of training. However, evidence now shows that voluntary movement and cardiovascular function can be improved from the first time epidural stimulation is used, if the stimulation parameters are specific for the function and person, which supports the role of epidural stimulation in improving function.
Access to new medical treatment for those requiring it cannot come soon enough. Experimental therapies are typically expensive and not covered by health care. Rigorous and sufficient testing is required before treatments become standard practice and receive health care coverage. Epidural stimulation for improving function in SCI is a unique example because epidural stimulation technology has been used widely to treat intractable back pain in individuals without SCI. The benefit of this is that, if/when epidural stimulation for individuals with SCI is shown to be safe and effective, the move from experimental clinical practice could happen relatively quickly as a number of hurdles from regulatory bodies have already been overcome. That said, current barriers to accessing epidural stimulation noted in a survey study of doctors include a lack of strong evidence research showing benefits, a lack of guidelines for the right stimulation settings, and an inability to determine who will benefit from it.
In Canada, the cost for an institution to install an epidural stimulation system for back pain in those without spinal cord injury, which is a common procedure, was $21,595 CAD. The cost incurred by a Canadian citizen undergoing implantation in Canada is $0 as it is covered by publicly funded health care.
In the United States, the cost for an institution to install an epidural stimulation system for back pain in those without spinal cord injury ranged between $32,882 USD (Medicare) and $57,896 USD (Blue Cross Blue Shield). The cost incurred for American citizens in the US will vary widely depending on their insurance coverage.
In contrast, for individuals with SCI, an epidural stimulation system is reported to cost over $100,000 USD in Thailand, and higher in other countries. Prospective clients should be aware that the epidural stimulation offered by these clinics may not be the same as that in the research reported in this article.
The recommended course for those wishing to try epidural stimulation is to register in a clinical trial. Regardless, persons interested in pursuing surgery at a private clinic or registering for clinical trials will find it useful to refer to the clinical trial guidelines published by ICORD (https://icord.org/research/iccp-clinical-trials-information/) for information on what they should be aware of when considering having an epidural stimulator implanted. Research studies that involve epidural stimulation can be found by searching the clinicaltrials.gov database.
Overall, there is evidence that epidural stimulation can improve function and health after SCI in numerous ways. However, because of the invasive nature of epidural stimulator implantation, research in this area involves few participants, no control groups, and no randomization, so it is classified as weak evidence. It is therefore important to keep in mind that while these recent reports are encouraging, more rigorous studies with more participants are needed to confirm the benefits and risks of this treatment to determine its place in SCI symptom management.
Epidural stimulation is not “plug and play” technology. Each implanted device needs to be tailored to the spine of the recipient. Some individuals respond to certain stimulation settings while others may respond better to other settings. Furthermore, over time, the need to change stimulation settings or even reposition the implant to maintain effectiveness may be required. Extensive physical training appears to be required for epidural stimulation to be most effective in improving standing or walking. The additional benefit of epidural stimulation to walk training is not always clear from the literature.
For a review of how we assess evidence at SCIRE Community and advice on making decisions, please see SCIRE Community Evidence.
Parts of this page have been adapted from the SCIRE Project (Professional) “Spasticity”, “Bladder Management”, and “Pain Management” chapters:
Hsieh JTC, Connolly SJ, McIntyre A, Townson AF, Short C, Mills P, Vu V, Benton B, Wolfe DL (2016). Spasticity Following Spinal Cord Injury. In Eng JJ, Teasell RW, Miller WC, Wolfe DL, Townson AF, Hsieh JTC, Connolly SJ, Curt A, Mehta S, Sakakibara BM, editors. Spinal Cord Injury Rehabilitation Evidence. Version 6.0.
Available from: scireproject.com/evidence/rehabilitation-evidence/spasticity/
Hsieh J, McIntyre A, Iruthayarajah J, Loh E, Ethans K, Mehta S, Wolfe D, Teasell R. (2014). Bladder Management Following Spinal Cord Injury. In Eng JJ, Teasell RW, Miller WC, Wolfe DL, Townson AF, Hsieh JTC, Connolly SJ, Noonan VK, Loh E, McIntyre A, editors. Spinal Cord Injury Rehabilitation Evidence. Version 5.0: p 1-196.
Available from: scireproject.com/evidence/rehabilitation-evidence/bladder-management/
Mehta S, Teasell RW, Loh E, Short C, Wolfe DL, Benton B, Hsieh JTC (2016). Pain Following Spinal Cord Injury. In Eng JJ, Teasell RW, Miller WC, Wolfe DL, Townson AF, Hsieh JTC, Connolly SJ, Loh E, McIntyre A, Querée M, editors. Spinal Cord Injury Rehabilitation Evidence. Version 6.0: p 1-92.
Available from: scireproject.com/evidence/rehabilitation-evidence/pain-management/
International Neuromodulation Society. (2010). Neuromodulation: An Emerging Field.
Toossi, A., Everaert, D. G., Azar, A., Dennison, C. R., & Mushahwar, V. K. (2017). Mechanically Stable Intraspinal Microstimulation Implants for Human Translation. Annals of Biomedical Engineering, 45(3), 681–694. Retrieved from http://link.springer.com/10.1007/s10439-016-1709-0
Lu, D. C., Edgerton, V. R., Modaber, M., AuYong, N., Morikawa, E., Zdunowski, S., … Gerasimenko, Y. (2016a). Engaging Cervical Spinal Cord Networks to Reenable Volitional Control of Hand Function in Tetraplegic Patients. Neurorehabilitation & Neural Repair, 30(10), 951–962. Retrieved from http://www.ncbi.nlm.nih.gov/pubmed/27198185
Lu, D. C., Edgerton, V. R., Modaber, M., AuYong, N., Morikawa, E., Zdunowski, S., … Gerasimenko, Y. (2016b). Engaging Cervical Spinal Cord Networks to Reenable Volitional Control of Hand Function in Tetraplegic Patients. Neurorehabilitation & Neural Repair, 30(10), 951–962.
Moore, D. M., & McCrory, C. (2016). Spinal cord stimulation. BJA Education, 16(8), 258–263. Retrieved from https://linkinghub.elsevier.com/retrieve/pii/S2058534917300975
Wolter, T. (2014). Spinal cord stimulation for neuropathic pain: current perspectives. Journal of Pain Research, 7, 651–663.
Eldabe, S., Buchser, E., & Duarte, R. V. (2015). Complications of Spinal Cord Stimulation and Peripheral Nerve Stimulation Techniques: A Review of the Literature. Pain Medicine, 17(2), pnv025. Retrieved from https://academic.oup.com/painmedicine/article-lookup/doi/10.1093/pm/pnv025
Taccola, G., Barber, S., Horner, P. J., Bazo, H. A. C., & Sayenko, D. (2020). Complications of epidural spinal stimulation: lessons from the past and alternatives for the future. Spinal Cord, 58(10), 1049–1059. Retrieved from http://dx.doi.org/10.1038/s41393-020-0505-8
Herrity, A. N., Williams, C. S., Angeli, C. A., Harkema, S. J., & Hubscher, C. H. (2018). Lumbosacral spinal cord epidural stimulation improves voiding function after human spinal cord injury. Scientific Reports, 8(1), 1–11. Retrieved from http://dx.doi.org/10.1038/s41598-018-26602-2
Herrity, April N., Aslan, S. C., Ugiliweneza, B., Mohamed, A. Z., Hubscher, C. H., & Harkema, S. J. (2021). Improvements in Bladder Function Following Activity-Based Recovery Training With Epidural Stimulation After Chronic Spinal Cord Injury. Frontiers in Systems Neuroscience, 14(January), 1–14.
Hubscher, C. H., Herrity, A. N., Williams, C. S., Montgomery, L. R., Willhite, A. M., Angeli, C. A., & Harkema, S. J. (2018). Improvements in bladder, bowel and sexual outcomes following task-specific locomotor training in human spinal cord injury. Plos One, 1–26.
Darrow, D., Balser, D., Netoff, T. I., Krassioukov, A., Phillips, A., Parr, A., & Samadani, U. (2019). Epidural Spinal Cord Stimulation Facilitates Immediate Restoration of Dormant Motor and Autonomic Supraspinal Pathways after Chronic Neurologically Complete Spinal Cord Injury. Journal of Neurotrauma, 2336, neu.2018.6006. Retrieved from https://www.liebertpub.com/doi/10.1089/neu.2018.6006
Beck, L., Veith, D., Linde, M., Gill, M., Calvert, J., Grahn, P., … Zhao, K. (2020). Impact of long-term epidural electrical stimulation enabled task-specific training on secondary conditions of chronic paraplegia in two humans. Journal of Spinal Cord Medicine, 0(0), 1–6. Retrieved from https://doi.org/10.1080/10790268.2020.1739894
Terson de Paleville, D. G. L., Harkema, S. J., & Angeli, C. A. (2019). Epidural stimulation with locomotor training improves body composition in individuals with cervical or upper thoracic motor complete spinal cord injury: A series of case studies. The Journal of Spinal Cord Medicine, 42(1), 32–38.
Guan, Y. (2012). Spinal cord stimulation: neurophysiological and neurochemical mechanisms of action. Current Pain and Headache Reports, 16(3), 217–225.
Marchand, S. (2015). Spinal cord stimulation analgesia. PAIN, 156(3), 364–365.
Tasker, R. R., DeCarvalho, G. T., & Dolan, E. J. (1992). Intractable pain of spinal cord origin: clinical features and implications for surgery. Journal of Neurosurgery.
Cioni, B., Meglio, M., Pentimalli, L., & Visocchi, M. (1995). Spinal cord stimulation in the treatment of paraplegic pain. Journal of Neurosurgery, 82(1), 35–39.
Warms, C. A., Turner, J. A., Marshall, H. M., & Cardenas, D. D. (2002). Treatments for chronic pain associated with spinal cord injuries: many are tried, few are helpful. Clinical Journal of Pain, 18(3), 154–163.
Reck, T. A., & Landmann, G. (2017). Successful spinal cord stimulation for neuropathic below-level spinal cord injury pain following complete paraplegia: a case report. Spinal Cord Series and Cases, 3, 17049.
Hachmann, J. T., Grahn, P. J., Calvert, J. S., Drubach, D. I., Lee, K. H., & Lavrov, I. A. (2017). Electrical Neuromodulation of the Respiratory System After Spinal Cord Injury. Mayo Clinic Proceedings, 92(9), 1401–1414. Retrieved from http://www.ncbi.nlm.nih.gov/pubmed/28781176
DiMarco, A. F., Kowalski, K. E., Geertman, R. T., & Hromyak, D. R. (2006). Spinal cord stimulation: a new method to produce an effective cough in patients with spinal cord injury. American Journal of Respiratory and Critical Care Medicine, 173(12), 1386–1389.
DiMarco, A. F., Kowalski, K. E., Geertman, R. T., & Hromyak, D. R. (2009). Lower thoracic spinal cord stimulation to restore cough in patients with spinal cord injury: results of a National Institutes of Health-sponsored clinical trial. Part I: methodology and effectiveness of expiratory muscle activation. Archives of Physical Medicine & Rehabilitation, 90(5), 717–725.
Harkema, S. J., Wang, S., Angeli, C. A., Chen, Y., Boakye, M., Ugiliweneza, B., & Hirsch, G. A. (2018). Normalization of Blood Pressure With Spinal Cord Epidural Stimulation After Severe Spinal Cord Injury. Frontiers in Human Neuroscience, 12, 83.
DiMarco, A. F., Kowalski, K. E., Hromyak, D. R., & Geertman, R. T. (2014). Long-term follow-up of spinal cord stimulation to restore cough in subjects with spinal cord injury. The Journal of Spinal Cord Medicine, 37(4), 380–388.
Harkema, S., Gerasimenko, Y., Hodes, J., Burdick, J., Angeli, C., Chen, Y., … Edgerton, V. R. (2011). Effect of epidural stimulation of the lumbosacral spinal cord on voluntary movement, standing, and assisted stepping after motor complete paraplegia: A case study. The Lancet, 377(9781), 1938–1947.
Darrow, D., Balser, D., Netoff, T. I., Krassioukov, A., Phillips, A., Parr, A., & Samadani, U. (2019). Epidural Spinal Cord Stimulation Facilitates Immediate Restoration of Dormant Motor and Autonomic Supraspinal Pathways after Chronic Neurologically Complete Spinal Cord Injury. Journal of Neurotrauma, 2336, neu.2018.6006. Retrieved from https://www.liebertpub.com/doi/10.1089/neu.2018.600
Nagel, S. J., Wilson, S., Johnson, M. D., Machado, A., Frizon, L., Chardon, M. K., … Howard, M. A. 3rd. (2017). Spinal Cord Stimulation for Spasticity: Historical Approaches, Current Status, and Future Directions. Neuromodulation: Journal of the International Neuromodulation Society, 20(4), 307–321.
Dekopov, A. V., Shabalov, V. A., Tomsky, A. A., Hit, M. V., & Salova, E. M. (2015). Chronic spinal cord stimulation in the treatment of cerebral and spinal spasticity. Stereotactic and Functional Neurosurgery.
Dimitrijevic, M. R., Illis, L. S., Nakajima, K., Sharkey, P. C., & Sherwood, A. M. (1986). Spinal cord stimulation for the control of spasticity in patients with chronic spinal cord injury: II. Neurophysiologic observations. Central Nervous System Trauma, 3(2), 145–152. Retrieved from http://ovidsp.ovid.com/ovidweb.cgi?T=JS&CSC=Y&NEWS=N&PAGE=fulltext&D=med2&AN=3490313
Midha, M., & Schmitt, J. K. (1998). Epidural spinal cord stimulation for the control of spasticity in spinal cord injury patients lacks long-term efficacy and is not cost-effective. Spinal Cord, 36(3), 190–192. Retrieved from https://www.nature.com/articles/3100532
Barolat, G., Singh-Sahni, K., Staas, W. E. J., Shatin, D., Ketcik, B., & Allen, K. (1995). Epidural spinal cord stimulation in the management of spasms in spinal cord injury: a prospective study. Stereotactic & Functional Neurosurgery, 64(3), 153–164.
Dekopov, A. V., Shabalov, V. A., Tomsky, A. A., Hit, M. V., & Salova, E. M. (2015). Chronic spinal cord stimulation in the treatment of cerebral and spinal spasticity. Stereotactic and Functional Neurosurgery.
Pinter, M. M., Gerstenbrand, F., & Dimitrijevic, M. R. (2000). Epidural electrical stimulation of posterior structures of the human lumbosacral cord: 3. Control Of spasticity. Spinal Cord, 38(9), 524–531. Retrieved from https://www.nature.com/articles/3101040
Edgerton, V. R., & Harkema, S. (2011). Epidural stimulation of the spinal cord in spinal cord injury: current status and future challenges. Expert Review of Neurotherapeutics, 11(10), 1351–1353. Retrieved from http://ovidsp.ovid.com/ovidweb.cgi?T=JS&CSC=Y&NEWS=N&PAGE=fulltext&D=med7&AN=21955190
Harkema, S. J., Gerasimenko, Y., Hodes, J., Burdick, J., Angeli, C., Chen, Y., … Edgerton, V. R. (2011). Supplementary index: Effect of epidural stimulation of the lumbosacral spinal cord on voluntary movement, standing, and assisted stepping after motor complete paraplegia: A case study. The Lancet, 377(9781), 1938–1947. Retrieved from http://www.ncbi.nlm.nih.gov/pubmed/21601270
Bloom, O., Wecht, J. M., Legg Ditterline, B. E., Wang, S., Ovechkin, A. V., Angeli, C. A., … Harkema, S. J. (2020). Prolonged Targeted Cardiovascular Epidural Stimulation Improves Immunological Molecular Profile: A Case Report in Chronic Severe Spinal Cord Injury. Frontiers in Systems Neuroscience, 14(October), 1–11.
Lu, D. C., Edgerton, V. R., Modaber, M., AuYong, N., Morikawa, E., Zdunowski, S., … Gerasimenko, Y. (2016a). Engaging Cervical Spinal Cord Networks to Reenable Volitional Control of Hand Function in Tetraplegic Patients. Neurorehabilitation & Neural Repair, 30(10), 951–962. Retrieved from http://www.ncbi.nlm.nih.gov/pubmed/27198185
Rejc, E., Angeli, C. A., Bryant, N., & Harkema, S. J. (2017). Effects of Stand and Step Training with Epidural Stimulation on Motor Function for Standing in Chronic Complete Paraplegics. Journal of Neurotrauma, 34, 1787–18023. Retrieved from www.liebertpub.com
Angeli, C. A., Edgerton, V. R., Gerasimenko, Y. P., & Harkema, S. J. (2014). Altering spinal cord excitability enables voluntary movements after chronic complete paralysis in humans. Brain, 137(Pt 5), 1394–1409. Retrieved from https://www.ncbi.nlm.nih.gov/pmc/articles/PMC3999714/
Peña Pino, I., Hoover, C., Venkatesh, S., Ahmadi, A., Sturtevant, D., Patrick, N., Freeman, D., Parr, A., Samadani, U., Balser, D., Krassioukov, A., Phillips, A., Netoff, T. I., & Darrow, D. (2020). Long-Term Spinal Cord Stimulation After Chronic Complete Spinal Cord Injury Enables Volitional Movement in the Absence of Stimulation. Frontiers in systems neuroscience, 14, 35. https://doi.org/10.3389/fnsys.2020.00035
Grahn, P. J., Lavrov, I. A., Sayenko, D. G., Straaten, M. G. Van, Gill, M. L., Strommen, J. A., … Lee, K. H. (2017). Enabling Task-Specific Volitional Motor Functions via Spinal Cord Neuromodulation in a Human with Paraplegia. Mayo Clinic Proceedings, 92(4), 544–554. Retrieved from http://dx.doi.org/10.1016/j.mayocp.2017.02.014
Harkema, S. J., Gerasimenko, Y., Hodes, J., Burdick, J., Angeli, C., Chen, Y., … Edgerton, V. R. (2011). Supplementary index: Effect of epidural stimulation of the lumbosacral spinal cord on voluntary movement, standing, and assisted stepping after motor complete paraplegia: A case study. The Lancet, 377(9781), 1938–1947. Retrieved from http://www.ncbi.nlm.nih.gov/pubmed/21601270
Rejc, E., Angeli, C. A., Atkinson, D., & Harkema, S. J. (2017). Motor recovery after activity-based training with spinal cord epidural stimulation in a chronic motor complete paraplegic. Scientific Reports, 7(1), 13476. Retrieved from www.nature.com/scientificreports
Rejc, E., Angeli, C., & Harkema, S. (2015). Effects of Lumbosacral Spinal Cord Epidural Stimulation for Standing after Chronic Complete Paralysis in Humans. PLoS ONE [Electronic Resource], 10(7), e0133998. Retrieved from http://ovidsp.ovid.com/ovidweb.cgi?T=JS&CSC=Y&NEWS=N&PAGE=fulltext&D=med8&AN=26207623
Grahn, P. J., Lavrov, I. A., Sayenko, D. G., Straaten, M. G. Van, Gill, M. L., Strommen, J. A., … Lee, K. H. (2017). Enabling Task-Specific Volitional Motor Functions via Spinal Cord Neuromodulation in a Human with Paraplegia. Mayo Clinic Proceedings, 92(4), 544–554. Retrieved from http://dx.doi.org/10.1016/j.mayocp.2017.02.014
Gill, M. L., Grahn, P. J., Calvert, J. S., Linde, M. B., Lavrov, I. A., Strommen, J. A., … Zhao, K. D. (2018). Neuromodulation of lumbosacral spinal networks enables independent stepping after complete paraplegia. Nature Medicine, 24(11), 1677–1682. Retrieved from https://doi.org/10.1038/s41591-018-0175-7
Angeli, C. A., Boakye, M., Morton, R. A., Vogt, J., Benton, K., Chen, Y., … Harkema, S. J. (2018). Recovery of Over-Ground Walking after Chronic Motor Complete Spinal Cord Injury. New England Journal of Medicine, 379(13), 1244–1250. Retrieved from http://ovidsp.ovid.com/ovidweb.cgi?T=JS&CSC=Y&NEWS=N&PAGE=fulltext&D=medl&AN=30247091
van de Port, I. G., Kwakkel, G., & Lindeman, E. (2008). Community ambulation in patients with chronic stroke: How is it related to gait speed? Journal of Rehabilitation Medicine, 40(1), 23–27.
Wagner, F. B., Mignardot, J.-B., Le Goff-Mignardot, C. G., Demesmaeker, R., Komi, S., Capogrosso, M., … Courtine, G. (2018). Targeted neurotechnology restores walking in humans with spinal cord injury. Nature, 563(7729), 65–71. Retrieved from http://www.nature.com/articles/s41586-018-0649-2
Angeli, C. A., Edgerton, V. R., Gerasimenko, Y. P., & Harkema, S. J. (2014). Altering spinal cord excitability enables voluntary movements after chronic complete paralysis in humans. Brain, 137(Pt 5), 1394–1409. Retrieved from https://www.ncbi.nlm.nih.gov/pmc/articles/PMC3999714/
Carhart, M. R., He, J., Herman, R., D’Luzansky, S., & Willis, W. T. (2004). Epidural spinal-cord stimulation facilitates recovery of functional walking following incomplete spinal-cord injury. IEEE Transactions on Neural Systems & Rehabilitation Engineering, 12(1), 32–42. Retrieved from http://ovidsp.ovid.com/ovidweb.cgi?T=JS&CSC=Y&NEWS=N&PAGE=fulltext&D=med5&AN=15068185
Harkema, S. J., Wang, S., Angeli, C. A., Chen, Y., Boakye, M., Ugiliweneza, B., & Hirsch, G. A. (2018). Normalization of Blood Pressure With Spinal Cord Epidural Stimulation After Severe Spinal Cord Injury. Frontiers in Human Neuroscience, 12, 83.
Legg Ditterline, B. E., Aslan, S. C., Wang, S., Ugiliweneza, B., Hirsch, G. A., Wecht, J. M., & Harkema, S. (2020). Restoration of autonomic cardiovascular regulation in spinal cord injury with epidural stimulation: a case series. Clinical Autonomic Research, (0123456789), 2–5. Retrieved from https://doi.org/10.1007/s10286-020-00693-2
Solinsky, R., Specker-Sullivan, L., & Wexler, A. (2020). Current barriers and ethical considerations for clinical implementation of epidural stimulation for functional improvement after spinal cord injury. Journal of Spinal Cord Medicine, 43(5), 653–656.
Kumar, K., & Bishop, S. (2009). Financial impact of spinal cord stimulation on the healthcare budget: a comparative analysis of costs in Canada and the United States. Journal of Neurosurgery: Spine.
Author: Sharon Jang | Reviewer: Tova Plashkes | Published: 24 September 2020 | Updated: 7 December 2021
This page provides an overview of how spinal cord injury (SCI) affects breathing and coughing, and the acute treatments used to address these issues.
Key Points
The breathing process: During inhalation, the diaphragm (dark pink) moves down and the ribs expand. During exhalation, the diaphragm moves up and the ribs contract.1
The respiratory system is responsible for helping you breathe in (inhaling) and out (exhaling). Breathing is done through the nose and mouth, although the nose is more often used. One reason the nose is used more often is because it acts as a filter for debris, which protects the lungs. Coughing is another important part of the respiratory system, as it helps to clear mucus from your lungs and airways.
Many muscles help with respiratory functions. The muscles used for inhaling are controlled from the spinal nerves of the neck (C3-C5 primarily), with some help from the nerves of the lower neck and thorax (C6-T12). Inhaling is mostly facilitated by your diaphragm, which is a large dome shaped muscle underneath the lungs. When you breathe in, your diaphragm lowers and the space in your chest increases, pulling air into the lungs. When you exhale, your ribs move back in and the diaphragm moves back up.
Breathing during exercise and coughing requires extra effort. To help, the abdominal muscles (over your belly) and the intercostal muscles (which help to squeeze the ribs) are activated, allowing for a stronger inhalation and a forceful cough.
When someone is unable to breathe or cough by themselves, their function, independence, and health are affected. These respiratory complications arise in 36-83% of individuals after SCI. This is due to disruption of the breathing nerves after an injury, and to secondary complications of an SCI, such as spasticity. After an SCI, breathing muscles may be partially or completely affected, depending on the completeness of the injury. However, breathing ability may improve over time.
Many muscles are required for breathing. The diagram above shows the main muscles of breathing, and the sections of the spinal cord that innervate them.2
The chances of experiencing respiratory complications depend on a variety of factors, including:
Refer to our page on Evidence Rating for more information on conflicting evidence.
After injury, the amount of air that can be inhaled and exhaled are significantly reduced for people with cervical and higher thoracic (neck and upper back) level injuries. More specifically, the amount of air that can fill the lungs (known as the total lung capacity) is reduced to 60-80% of normal values. Additionally, the amount of air that can be exhaled after the biggest breath in (known as the vital capacity) is reduced to 50-80% of normal values. This contributes to inefficient breathing that may be tiresome and difficult. In addition, a lower vital capacity can impact voice volume, making it difficult to speak at louder volumes.
An SCI can affect the lung itself. The main change is a reduction in lung compliance, or the lung’s ability to stretch and expand. As a result, the lung does not spring back “closed” after being open. In addition, the compliance of the rib cage (chest wall) may also decrease, causing the chest to become rigid in individuals with tetraplegia. Reduced compliance results in a decreased ability to take a deep breath independently or with the help of a breathing bag or ventilator.
Coughing is important to keep the airway and lungs clear from mucus. This is because a build up of mucus can collapse the lungs, and mucus in the airways can result in infection. In order to perform a cough, one needs to inhale deeply then have a forceful exhale while a structure called the glottis closes the entrance to the windpipe. The intercostal muscles and the abdominal muscles assist with the ability to increase the force of exhaled air. As these muscles are innervated by nerves in the chest region, individuals with spinal cord injuries may have an impaired coughing function. Cough function may be completely absent in some individuals, while others may have limited or ineffective coughing abilities.
Soon after injury, it is common for individuals with high-level SCI to produce a lot of mucus in their lungs and have smaller airways deep in the lung. The lungs are also very irritable to stimuli like too much suctioning of mucus, or smoking. This may be due to the increased influence of the parasympathetic nervous system after SCI. In people with acute tetraplegia, it has been reported that an excess of up to 1 liter of mucus is produced each day. In combination with an inability to cough, this excess production of mucus can result in a buildup of fluid in the lungs and airway.
Although swallowing is important for eating, it is also important for clearing the throat to prevent food, drink, stomach contents, or saliva from entering the lungs (also known as aspiration). After SCI, the risk of aspiration increases as:
The lack of effective swallowing can cause mucus to collect in your airway. Over time, the stagnant mucus can encourage the growth of bacteria, which may travel down to your lungs and potentially result in pneumonia.
Efficient removal of mucus from the airways is important to prevent choking and lung infections, especially when independent coughing is difficult. Although research on the topic of secretion removal techniques is scarce, one study with moderate evidence showed that manual removal techniques combined with mechanical removal techniques are effective in SCI early after injury. Different techniques are outlined below:
Certain body positions can use gravity to drain mucus towards the throat to be excreted easier. For example, laying on your side with your feet elevated can help drain the lower lung. In order for these positions to be effective, your body must be positioned in specific angles. Refer to your healthcare professional for more information. To facilitate breakup and movement of the mucus buildup in the lungs, postural drainage can be paired with applying pressure to the chest (chest percussion) or shaking the chest (vibration).
Physical pressure is applied to the chest or abdomen right before expiration to help the individual breathe out. This can be done on yourself or by a trained family member or caregiver.
There are machines that help loosen secretions, clear mucus, and can trigger a cough. They work by delivering a deep breath by pushing air into the lungs, then facilitate exhalation by sucking the air out.
A tube can be inserted through the mouth or tracheostomy site to suction mucus that is stuck in the upper airways. Suctioning may also reflexively trigger a cough.
Weak inspiratory muscles can result in breathlessness. Like exercise training, inspiratory muscle strength and endurance can increase with training and decrease bouts of shortness of breath (dyspnea) and coughing. Inspiratory muscle training involves using devices that create resistance when breathing in.
Resistive trainers have adjustable settings that allow individualized training programs.5
Refer to our article on Inspiratory Muscle Training for more information!
People with tetraplegia have increased sensitivity of their airways, resulting in more frequent narrowing. To treat this, a family of drugs called bronchodilators can be used to enlarge the airways for air to pass through with more ease. The use of bronchodilators is supported by multiple (weak evidence) studies, which have found that bronchodilators can help improve expiration among individuals with tetraplegia. There is also one strong evidence study that indicates that the bronchodilator salmeterol can improve both respiratory functioning and the strength of breathing muscles. While bronchodilators can help positively influence respiratory functioning, their use carries a potential negative side effect of thickening mucus.
Mechanical ventilation, or machine assisted breathing, is becoming more common as there has been an increase in the number of people who survive cervical level injuries over the past 40 years. Mechanical ventilation is used by people who are unable to breathe independently, often right after injury. The machine works by pushing air into the lungs until a pre-set volume or pressure is reached. Once the pre-set value has been met, the machine stops pushing air in and the air is exhaled by the person.
In general, there are two forms of mechanical ventilation: a non-invasive approach where a mask is placed over the mouth and nose (known as a Bilevel Positive Airway Pressure (BiPAP) or Continuous Positive Airway Pressure (or CPAP)), or an invasive approach where a tube is inserted into the windpipe via the mouth and throat (intubation) or directly into the windpipe through a surgical incision (tracheostomy). Intubation or tracheostomy is used in more severe cases to ensure air gets to the lungs and that mucus is filtered out of the lung. Factors that increase the chances of requiring invasive mechanical ventilation include having a complete injury, having a higher level of injury, or having a compound injury Whenever possible, the healthcare team tries to help people breathe on their own and “weans” the person off the ventilator if possible.
The process of intubation consists of running a tube into the trachea either through the nose or mouth. This process is completed as soon as someone is in respiratory distress, which is normally at the scene of the accident or upon admission to the hospital. Intubation is often used for a short term (i.e., less than 10 days), as prolonged use can lead to severe weakness of breathing muscles, pneumonia, more difficulty in breathing, mobility limitations, prolonged ventilator weaning, and can make lung and mouth hygiene difficult.
A tracheostomy is a surgical procedure that involves placing a tube through an opening in the throat and windpipe. This creates a pathway for air delivery from a ventilator and to facilitate secretion removal. However, after the tracheostomy tube is taken out, speaking and eating may be difficult as the throat muscles become weakened and uncoordinated.
A tracheostomy tube is inserted into the throat through a surgical hole.6
A tracheostomy is performed in the event that breathing support is required for a minimum of 3 weeks. Individuals who may require a tracheostomy (weak to moderate evidence) include: having a complete or higher level of injury, having a complete injury or a lower AIS motor grade, and old age.
Once an individual is able to independently breathe, the tube is removed from the windpipe. Weak evidence suggests that tube removal is more successful in individuals with certain characteristics:
Continuous Positive Airway Pressure (CPAP) is a form of mechanical ventilation commonly used to address obstructive sleep apnea. Sleep apnea occurs when breathing ceases in short bouts during sleep, and can result in feeling tired during the day. CPAP machines are used to manage this condition by acting as a “pneumatic splint”, keeping airways open during sleep.
Loss of independent breathing and cough function can lead to secondary respiratory issues. These issues need to be medically addressed, as they may be life-threatening if left untreated.
A collapsed lung in comparison to a healthy lung.7
A condition where a part of the lung becomes partially or fully collapsed due to a lack of air. This results in a reduced ability to exchange oxygen and carbon dioxide. When the body does not get enough oxygen, organs will start to shut down. Atelectasis can result from anything that prevents the lungs from fully expanding, including:
Pressure from outside the lungs, which can result in the inability to fully inflate. This external pressure may stem from fluid or air, abdominal organs, or external hardware such as a brace.
Pneumonia is a medical name for a lung infection. After an SCI, several factors make pneumonia very common:
Requiring a ventilator, suctioning (removal of secretions with a special machine), or a tracheostomy may often be necessary, but tends to introduce bacteria despite best efforts at hygiene and air filtration. The risk of getting pneumonia increases if someone:
Refer to our article on Infectious Respiratory Conditions for more information!
A pulmonary embolism occurs when a clot (red groups) gets caught in the lungs.8
A pulmonary embolism is a blockage of an artery in the lungs by a blood clot that has moved from elsewhere in the body through the bloodstream (embolism). As a result of paralysis or immobility, a blockage may develop in a vein, often in the lower leg. This is called a blood clot, or deep vein thrombosis. The clot may travel to the lung and block blood vessels, resulting in sudden shortness of breath. The prevalence of this condition is highest within the first three months of injury. Weak evidence suggests that pulmonary embolisms occur in a range of 1.25% to 4.5% of people with SCI in the first 90 days. However, pulmonary embolisms have been considered to occur rarely after the first three months of SCI, and have decreased significantly due to preventative measures (e.g., blood thinners). That said, weak evidence suggests that pulmonary embolisms may still be an issue in chronic SCI, but may not be severe enough to cause any symptoms.
Pulmonary edema is a build-up of fluid in the lung. This often occurs in early stages following injury. It can affect as much as 50% of individuals with acute tetraplegia. There are several causes, with the most common being excess fluids given to people with SCI. After an SCI, blood pressure may drop to very low levels. Depending on the cause and type of injury, this may be due to blood loss from a traumatic injury, or impairment of nerves that keep blood pressure at its normal level with a cervical or high thoracic injury. As a result, a lot of fluids are given to patients to help their blood pressure recover.
Respiratory failure occurs when the respiratory system is damaged to the extent where the body does not get enough oxygen and is unable to get rid of carbon dioxide. Oxygen levels in the body may drop to critically low values and carbon dioxide, which is poisonous at very high levels, builds up. The risk of a respiratory failure increases with higher levels of injury, and most commonly occurs in acute SCI. This usually results in the need for mechanical ventilation.
Getting vaccinated is one of the ways to prevent secondary complications such as pneumonia.9
Prevention is important to avoid getting respiratory illnesses when you have an SCI. Some things you can do to stay as healthy as possible include:
Equipment used for lung volume recruitment.10
Upon returning to the community, common secretion removal techniques include glossopharyngeal breathing and the use of lung volume recruitment (LVR) bags with an assisted cough. Glossopharyngeal breathing (or frog breathing) is a technique that is used to get a deeper breath. This is done by rapidly taking “gulps” of breaths one after the other, followed by exhaling. This can help create a cough, or facilitate assisted coughing.
LVR, or “breath stacking” is done with an LVR kit which consists of a resuscitation bag connected with a flexible tube to a mouth piece with a one-way valve. The individual will inhale the most they can, and once this point is reached, a clinician (or second person) will squeeze the bag to “stack” breaths to fully inflate the lungs. This allows the individual to breathe more air than they are able to themselves, and to exhale more air more quickly to produce an improved cough. This also can help with maintaining chest mobility and flexibility.
Exercise training involving arm and leg movements can improve muscle strength and cardiovascular endurance. Breathing muscles are also challenged with exercise and may become stronger with exercise. This increase in strength can help decrease the effort of breathing at rest and with functional activity, like transfers. An example of a method of exercise training for individuals with higher levels of injuries include the use of a Functional Electrical Stimulation (FES) bike. Other exercises like arm cycling or strengthening exercises are commonly prescribed by a physiotherapist or health care professional. While exercise can help strengthen respiratory muscles, low-moderate evidence studies debate whether lung volumes are impacted. This is to say, exercising may help make breathing feel easier, but it is unknown whether the amount of air you can take into your lungs is affected. High intensity exercise three times per week for six weeks has shown to significantly improve respiratory function. However, standard guidelines for high intensity exercise have not yet been established.
An abdominal binder wrapped around the abdomen to correct the positioning of the diaphragm.12
Refer to our article on Functional Electrical Stimulation for more information!
Girdles or abdominal binders are garments that apply pressure around the abdominal area to help keep the diaphragm in an optimal position. Abdominal binders are also used for managing orthostatic hypotension and blood pooling. Although there may be short-term improvements when using a girdle or binder, more research is needed in determining their long-term utility.
Refer to our article on Abdominal binders for more information!
For people who are ventilator dependent, various electrical stimulation techniques are available to assist with breathing. This includes phrenic nerve stimulation/diaphragm pacing, abdominal electrical stimulation, and epidural stimulation.
The diaphragm is the main muscle responsible for breathing and is activated by the phrenic nerve to contract. After SCI, the connection between the brain and the phrenic nerve is disrupted, which contributes to impaired breathing. Researchers have been looking at ways we can stimulate this nerve to reactivate the diaphragm through a process called phrenic nerve stimulation. This process involves surgically implanting electrodes and a receiver close to the phrenic nerve, either in the chest or the neck, and a receiver in the chest wall. This device is controlled with an external remote and antenna (which is used to connect to the electrode receiver).
For phrenic nerve stimulation to work, the diaphragm must have normal function, and the phrenic nerve needs to be intact (i.e., sends a signal when stimulated). As a result, individuals who have a C3, C4, or C5 level injury may not be eligible as they often have impaired phrenic nerve function. It is important to note that this procedure can only facilitate inspiratory functions, but not expiratory. As a result, an individual who receives phrenic nerve stimulation may not require mechanical ventilation, but will still require assistance with coughing and clearing secretions. Tracheostomies and mechanical ventilation are often still used in combination with phrenic nerve stimulation as a back-up.
Some weak evidence supports the use of phrenic nerve stimulation. One study found that diaphragm pacemakers have better results with long term implantation (i.e., 6.3 years in the study). Another study showed that diaphragmatic paces can improve survival rates, decrease the cost of care, improve the quality of speech, increase rates of social participation, and improve management of using a powered wheelchair. Many complications have been reported in the research in regards to using a phrenic nerve stimulator. These complications include wires breaking or getting displaced, device failure, inhaling food when eating, shoulder or abdominal pain, and infections.
As diaphragm pacing only helps with inhalation, limited research suggests that electrically stimulating the abdominal muscles helps with expiration and coughing. Ideally, the abdominal muscles would be used to support voluntary efforts to cough. There have been mixed findings on the effectiveness of stimulating the abdominal muscles to enhance cough. While some weak studies have found abdominal stimulation to improve cough, other weak evidence studies have found no noticeable changes. More research is required to determine the efficacy of stimulating the abdominal muscles to enhance cough in SCI.
Epidural stimulation is conducted through surgically implanting an electrode over the spinal cord. Once done, the electrode, which is controlled with a remote outside of the body, stimulates various parts of the spinal cord. Emerging research on epidural stimulation suggests that it may benefit respiratory function after SCI. By directly stimulating nerve cells in the spinal cord, weak evidence suggests that breathing muscles can be activated. The muscles are activated in a pattern that resembles normal breathing, while reducing fatigue. Additionally, weak evidence suggests that epidural stimulation can improve other respiratory functions including coughing and speaking.
Respiratory problems are common after SCI. The extent and type of these problems depend on the level of injury and completeness of injury. Both conservative and invasive options for managing respiratory health following an SCI are available. Due to impaired respiratory function, a variety of secondary complications to the lungs frequently occur after SCI. While prevention using proper respiratory hygiene is best, should you experience a secondary respiratory complication, a variety of management techniques can be applied. Some techniques are more common in the acute stages of SCI, while others are more suited to chronic SCI. It is best to discuss all treatment options with your health providers to find out which treatments are suitable for you.
Mullen E, Mirkowski M, Vu V, McIntyre A, Teasell RW. (2015). Respiratory Management during the Acute Phase of Spinal Cord Injury. In Eng JJ, Teasell RW, Miller WC, Wolfe DL, Townson AF, Hsieh JTC, Connolly SJ, Noonan VK, Loh E, McIntyre A, editors. Spinal Cord Injury Research Evidence. Version 5.0: p 1-50.
Available from: https://scireproject.com/evidence/acute-evidence/respiratory-management-during-acute-phase-of-spinal-cord-injury/
Warren, P. M., Awad, B. I., & Alilain, W. J. (2014). Drawing breath without the command of effectors: The control of respiration following spinal cord injury. Respiratory Physiology & Neurobiology. https://doi.org/10.1016/j.resp.2014.08.005
Lemons, V. R., & Wagner, F. C. (1994). Respiratory Complications After CSCI. In Spine (Vol. 19, Issue 20, pp. 2315–2320).
Romero-Ganuza, J., Gambarrutta, C., Merlo-Gonzalez, V. E., Marin-Ruiz, M. Á., Diez De La Lastra-Buigues, E., & Oliviero, A. (2011). Complications of tracheostomy after anterior cervical spine fixation surgery. American Journal of Otolaryngology – Head and Neck Medicine and Surgery, 32(5), 408–411. https://doi.org/10.1016/j.amjoto.2010.07.020
Romero, J., Vari, A., Gambarrutta, C., & Oliviero, A. (2009). Tracheostomy timing in traumatic spinal cord injury. European Spine Journal, 18(10), 1452–1457. https://doi.org/10.1007/s00586-009-1097-3
Aarabi, B., Harrop, J. S., Tator, C. H., Alexander, M., Dettori, J. R., Grossman, R. G., Fehlings, M. G., Mirvis, S. E., Shanmuganathan, K., Zacherl, K. M., Burau, K. D., Frankowski, R. F., Toups, E., Shaffrey, C. I., Guest, J. D., Harkema, S. J., Habashi, N. M., Andrews, P., Johnson, M. M., & Rosner, M. (2012). Predictors of pulmonary complications in blunt traumatic spinal cord injury. Journal of Neurosurgery: Spine, 17, 38–45.
Jain, N. B., Higgins, L. D., Katz, J. N., & Garshick, E. (2010). Association of shoulder pain with the use of mobility devices in persons with chronic spinal cord injury. PM and R, 2(10), 896–900. https://doi.org/10.1016/j.pmrj.2010.05.004
Anke, A., Aksnes, A. K., Stanghelle, J. K., & Hjeltnes, N. (1993). Lung volumes in tetraplegic patients according to cervical spinal cord injury level. Scandinavian Journal of Rehabilitation Medicine, 25(2), 73–77. http://www.ncbi.nlm.nih.gov/pubmed/8341994
Brown, R., DiMarco, A. F., Hoit, J. D., & Garshick, E. (2006). Respiratory dysfunction and management in spinal cord injury. Respiratory Care, 51(8), 853-68;discussion 869-70. http://www.ncbi.nlm.nih.gov/pubmed/16867197%0Ahttp://www.pubmedcentral.nih.gov/articlerender.fcgi?artid=PMC2495152
Berlly, M., & Shem, K. (2007). Respiratory management during the first five days after spinal cord injury. Journal of Spinal Cord Medicine, 30(4), 309–318. https://doi.org/10.1080/10790268.2007.11753946
Schilero, G. J., Spungen, A. M., Bauman, W. A., Radulovic, M., & Lesser, M. (2009). Pulmonary function and spinal cord injury. Respiratory Physiology and Neurobiology, 166(3), 129–141. https://doi.org/10.1016/j.resp.2009.04.002
Schilero, G.J., Grimm, D.R., Bauman, W.A., Lenner, R., Lesser, M. (2005). Assessment of airway caliber and bronchodilator responsiveness in subjects with spinal cord injury. Chest, 127(1), 149-155. http://dx.doi.org/10.1378/chest.127.1.149
Bhaskar, K. R., Brown, R., O’Sullivan, D. D., Melia, S., Duggan, M., & Reid, L. (1991). Bronchial Mucus Hypersecretion in Acute Quadriplegia: Macromolecular Yields and Glycoconjugate Composition. American Review of Respiratory Disease, 143(3), 640–648. https://doi.org/10.1164/ajrccm/143.3.640
Chaw, E., Shem, K., Castillo, K., Wong, S., & Chang, J. (2012). Dysphagia and associated respiratory considerations in cervical spinal cord injury. Topics in Spinal Cord Injury Rehabilitation, 18(4), 291–299. https://doi.org/10.1310/sci1804-291
Almenoff PL, Alexander LR, Spungen AM, Lesser MD, Bauman WA. Bronchodilatory effects of ipratropium bromide in patients with tetraplegia. Paraplegia 1995; 33: 274-7.
Spungen AM, Dicpinigaitis PV, Almenoff PL, Bauman WA. Pulmonary obstruction in individuals with cervical spinal cord lesions unmasked by bronchodilator administration. Paraplegia 1993;31:404-7.
Schilero GJ, Grimm D, Spungen AM, Lenner R, Lesser M. Bronchodilator responses to metaproterenol sulfate among subjects with spinal cord injury. J Rehabil Res Dev 2004; 41: 59-64.
Grimm DR, Schilero GJ, Spungen AM, Bauman WA, Lesser M. Salmeterol improves pulmonary function in persons with tetraplegia. Lung 2006; 184: 335–339.
Devivo, M. J. (2012). Epidemiology of traumatic spinal cord injury: Trends and future implications. Spinal Cord, 50(5), 365–372. https://doi.org/10.1038/sc.2011.178
Gregoretti, C., Olivieri, C., & Navalesi, P. (2005). Physiologic comparison between conventional mechanical ventilation and transtracheal open ventilation in acute traumatic quadriplegic patients. Critical Care Medicine, 33(5), 1114–1118. https://doi.org/10.1097/01.CCM.0000162559.74446.09
Como, J. J., Sutton, E. R. H., McCunn, M., Dutton, R. P., Johnson, S. B., Aarabi, B., & Scalea, T. M. (2005). Characterizing the need for mechanical ventilation following cervical spinal cord injury with neurologic deficit. Journal of Trauma – Injury, Infection and Critical Care, 59(4), 912–916. https://doi.org/10.1097/01.ta.0000187660.03742.a6
Montoto-Marqués, A., Trillo-Dono, N., Ferreiro-Velasco, M. E., Salvador-De La Barrera, S., Rodriguez-Sotillo, A., Mourelo-Fariña, M., Galeiras-Vázquez, R., & Meijide-Failde, R. (2018). Risks factors of mechanical ventilation in acute traumatic cervical spinal cord injured patients. Spinal Cord, 56(3), 206–211. https://doi.org/10.1038/s41393-017-0005-7
Seidl, R. O., Wolf, D., Nusser-Müller-Busch, R., & Niedeggen, A. (2010). Airway management in acute tetraplegics: A retrospective study. European Spine Journal, 19(7), 1073–1078. https://doi.org/10.1007/s00586-010-1328-7
Velmahos, G. C., Toutouzas, K., Chan, L., Tillou, A., Rhee, P., Murray, J., & Demetriades, D. (2003). Intubation after cervical spinal cord injury: to be done selectively or routinely? The American Surgeon, 69, 891–894. https://doi.org/10.1257/0002828041464551
Durbin, C. G., Bell, C. T., & Shilling, A. M. (2014). Elective intubation. Respiratory Care, 59(6), 825–849.
Shirawi, N., & Arabi, Y. (2005). Bench-to-bedside review: Early tracheostomy in critically ill trauma patients. In Critical Care (Vol. 10, Issue 1). BioMed Central Ltd. https://doi.org/10.1186/cc3828
Biering-Sorensen, M. (1992). Tracheostomy in spinal cord injured: frequency and follow up. 30, 656–660.
Ganuza, J. R., Forcada, A. G., Gambarrutta, C., Buigues, E. D. D. L. L., Gonzalez, V. E. M., Fuentes, F. P., & Luciani, A. A. (2011). Effect of technique and timing of tracheostomy in patients with acute traumatic spinal cord injury undergoing mechanical ventilation. Journal of Spinal Cord Medicine, 34(1), 76–84. https://doi.org/10.1179/107902610X12886261091875
McCully, B. H., Fabricant, L., Geraci, T., Greenbaum, A., Schreiber, M. A., & Gordy, S. D. (2014). Complete cervical spinal cord injury above C6 predicts the need for tracheostomy. American Journal of Surgery, 207(5), 668–669. https://doi.org/10.1016/j.amjsurg.2014.01.001
Yugué, I., Okada, S., Ueta, T., Maeda, T., Mori, E., Kawano, O., Takao, T., Sakai, H., Masuda, M., Hayashi, T., Morishita, Y., & Shiba, K. (2012). Analysis of the risk factors for tracheostomy in traumatic cervical spinal cord injury. Spine, 37(26), 1633–1638. https://doi.org/10.1097/BRS.0b013e31827417f1
Leelapattana, P., Fleming, J. C., Gurr, K. R., Bailey, S. I., Parry, N., & Bailey, C. S. (2012). Predicting the need for tracheostomy in patients with cervical spinal cord injury. Journal of Trauma and Acute Care Surgery, 73(4), 880–884. https://doi.org/10.1097/TA.0b013e318251fb34
Menaker, J., Kufera, J. A., Glaser, J., Stein, D. M., & Scalea, T. M. (2013). Admission ASIA motor score predicting the need for tracheostomy after cervical spinal cord injury. Journal of Trauma and Acute Care Surgery, 75(4), 629–634. https://doi.org/10.1097/TA.0b013e3182a12b86
O’Keeffe, T., Goldman, R. K., Mayberry, J. C., Rehm, C. G., & Hart, R. A. (2004). Tracheostomy after anterior cervical spine fixation. Journal of Trauma – Injury, Infection and Critical Care, 57(4), 855–860. https://doi.org/10.1097/01.TA.0000083006.48501.B2
Harrop, J. S., Sharan, A. D., Scheid, E. H., Vaccaro, A. R., & Przybylski, G. J. (2004). Tracheostomy placement in patients with complete cervical spinal cord injuries: American Spinal Injury Association Grade A. Journal of Neurosurgery, 100(Spine 1), 20–23.
Peterson, W., Charlifue, W., Gerhart, A., & Whiteneck, G. (1994). Two methods of weaning persons with quadriplegia from mechanical ventilators. Paraplegia, 32(2), 98–103. https://doi.org/10.1038/sc.1994.17
Kornblith, L. Z., Kutcher, M. E., Callcut, R. A., Redick, B. J., Hu, C. K., Cogbill, T. H., Baker, C. C., Shapiro, M. L., Burlew, C. C., Kaups, K. L., DeMoya, M. A., Haan, J. M., Koontz, C. H., Zolin, S. J., Gordy, S. D., Shatz, D. V, Paul, D. B., Cohen, M. J., & Western Trauma Association Study Group. (2013). Mechanical ventilation weaning and extubation after spinal cord injury: a Western Trauma Association multicenter study. The Journal of Trauma and Acute Care Surgery, 75(6), 1060–1069; discussion 1069-70. https://doi.org/10.1097/TA.0b013e3182a74a5b
Berlly, M., & Shem, K. (2007). Respiratory management during the first five days after spinal cord injury. Journal of Spinal Cord Medicine, 30(4), 309–318. https://doi.org/10.1080/10790268.2007.11753946
Garcia-Arguello, L. Y., O’Horo, J. C., Farrell, A., Blakney, R., Sohail, M. R., Evans, C. T., & Safdar, N. (2017). Infections in the spinal cord-injured population: a systematic review. Spinal Cord, 55(6), 526–534. https://doi.org/https://dx.doi.org/10.1038/sc.2016.173
Alabed, S., De Heredia, L. L., Naidoo, A., Belci, M., Hughes, R. J., & Meagher, T. M. (2015). Incidence of pulmonary embolism after the first 3 months of spinal cord injury. Spinal Cord, 53(11), 835–837. https://doi.org/10.1038/sc.2015.105
Aito, S., Pieri, A., D’Andrea, M., Marcelli, F., & Cominelli, E. (2002). Primary prevention of deep venous thrombosis and pulmonary embolism in acute spinal cord injured patients. Spinal Cord, 40(6), 300–303. https://doi.org/10.1038/sj.sc.3101298
Frisbie, J. H., & Sharma, G. V. R. K. (2012). The prevalence of pulmonary embolism in chronically paralyzed subjects: A review of available evidence. Spinal Cord, 50(6), 400–403. https://doi.org/10.1038/sc.2011.154
Sezer, N., Akkuş, S., & Uğurlu, F. G. (2015). Chronic complications of spinal cord injury. World Journal of Orthopaedics, 6(1), 24–33. https://doi.org/10.5312/wjo.v6.i1.24
DiMarco, A. F., Kowalski, K. E., Hromyak, D. R., & Geertman, R. T. (2014). Long-term follow-up of spinal cord stimulation to restore cough in subjects with spinal cord injury. The Journal of Spinal Cord Medicine, 37(4), 380–388. https://doi.org/10.1179/2045772313Y.0000000152
Carter, R. E. (1993). Experience with ventilator dependent patients ! In Paraplegia (Vol. 31). https://doi.org/10.1038/sc.1993.28
Hirschfeld, S., Exner, G., Luukkaala, T., & Baer, G. A. (2008). Mechanical ventilation or phrenic nerve stimulation for treatment of spinal cord injury-induced respiratory insufficiency. Spinal Cord, 46(11), 738–742. https://doi.org/10.1038/sc.2008.43
Esclarin, A., Bravo, P., Arroyo, O., Mazaira, J., Garrido, H., & Alcaraz, M. A. (1994). Tracheostomy ventilation versus diaphragmatic pacemaker ventilation in high spinal cord injury. Paraplegia, 32(10), 687–693. https://doi.org/10.1038/sc.1994.111
DiMarco, A. F., Kowalski, K. E., Geertman, R. T., & Hromyak, D. R. (2006). Spinal cord stimulation: a new method to produce an effective cough in patients with spinal cord injury. American Journal of Respiratory and Critical Care Medicine, 173(12), 1386–1389. https://doi.org/10.1164/rccm.200601-097CR
Hachmann, J. T., Grahn, P. J., Calvert, J. S., Drubach, D. I., Lee, K. H., & Lavrov, I. A. (2017). Electrical Neuromodulation of the Respiratory System After Spinal Cord Injury. Mayo Clinic Proceedings, 92(9), 1401–1414. https://doi.org/10.1016/j.mayocp.2017.04.011
Harkema, S. J., Wang, S., Angeli, C. A., Chen, Y., Boakye, M., Ugiliweneza, B., & Hirsch, G. A. (2018). Normalization of Blood Pressure With Spinal Cord Epidural Stimulation After Severe Spinal Cord Injury. Frontiers in Human Neuroscience, 12, 83. https://doi.org/10.3389/fnhum.2018.00083
Author: Sharon Jang | Reviewers: Phillip Popovich, Katherine Mifflin | Published: 2 September 2020 | Updated: 10 January 2025
The adverse effects of a spinal cord injury (SCI) on the respiratory and immune systems can increase the risk of getting an infectious respiratory condition. This page reviews the relationship between SCI and infectious respiratory conditions.
Key Points
The upper respiratory system (red) and lower respiratory system (blue).1
The respiratory system involves the lungs, and is responsible for breathing, coughing, and speaking. It consists of the upper respiratory tract (nose, mouth, and the throat (pharynx)), and the lower respiratory tract (voice box (larynx), airways (windpipe or trachea), and lungs). Breathing and coughing both depend on various muscles in the chest and neck. Changes to respiratory function after SCI depend on the level of injury and completeness of the injury. After SCI, especially high cervical level injury, some muscles required for breathing may be affected. These include:
The muscles used to breathe are mostly controlled by the upper parts of the spine.2
As a result, sustaining a higher-level injury may result in impaired respiratory function. Some of these changes include:
Despite these changes, many technologies and techniques are available that can assist with breathing and coughing. Moreover, the greatest amount of air you are able to blow out after taking your biggest breath in increases over time from injury.
For more information, refer to our article on Respiratory Changes After SCI.
The immune system is responsible for fighting infections and preventing illness. To keep our bodies healthy, the immune system does three main things:
The immune system is comprised of two main parts: the innate immune system and the adaptive (or acquired) immune system. The innate immune system refers to a non-specific line of defence (i.e., it acts against all germs in the same manner) that you are born with. It is often the first line of defense, which is made up of parts of your body that prevent germs from entering. This includes:
Many organs throughout the body contribute to producing cells required to keep you healthy.3
If germs manage to invade the body, the innate immune system is the first system to recognize them. The innate immune system will then trigger a general attack, such as inflammation and fever. This attack affects the entire body, and does not directly target the germ. Innate immune cells then enlist the help of the adaptive immune system.
The second line of defense involves the adaptive immune system, which initiates specific defences for each germ that enters your body. For example, the body will react differently to a virus that causes influenza versus one that causes measles. The main players in the second line of defense are various types of white blood cells. This includes: natural killer cells, which kill any cell that is not recognized as part of the body, lymphocytes, which help the body remember the invaders for the future and destroy them, and phagocytes, which help “eat” and break up invading organisms.
The cells that contribute to the first and second line of defence are produced in organs all over the body, including the bone marrow, spleen, lymph nodes, adrenal glands, tonsils, and thymus.
Spinal Cord Injury Immune Depression Syndrome (SCI-IDS) is a condition that weakens the immune system after SCI. Weak evidence suggests that SCI-IDS commonly occurs among those with acute SCI. That said, there is also early evidence that SCI-IDS can persist and be present in those with chronic (>1 year) SCI. Although researchers are unsure why the immune system is weakened after SCI, hypotheses have been made:
A natural killer cell. The number of these cells is decreased after SCI.4
Weak evidence suggests that immune system changes may occur regardless of level of injury. For example, the amount of natural killer cells are reduced in adults with SCI, regardless of level of injury, in comparison to an able-bodied population. This reduces the body’s ability to fight off germs, which may cause infections, disease, and illnesses. Moreover, early animal research suggests that individuals with SCI may be more susceptible to viruses, such as the flu due to impairments in the body’s immunity. However, it is important to note that these findings have not yet been replicated in humans.
Although the immune system recovers after acute SCI, some (weak) evidence suggests that lowered immunity may extend into the chronic stage of SCI. One study investigated the genes responsible for programming and developing immune cells. The authors found that the genes that normally create natural killer cells are reduced, resulting in lower amounts of these germ-fighting cells throughout the body. A second study supported these findings, as individuals with SCI were found to have lower amounts of natural killer cells in their blood compared to an able-bodied population.
Having an SCI in combination with a weakened immune system has many implications for secondary complications. For example, after SCI many individuals may have difficulties with or be unable to effectively void urine, which encourages the growth of bacteria. This, in combination with a weakened immune system, may explain why urinary tract infections (UTIs) are a common secondary complication of SCI. Although SCI complications and a weakened immune system may contribute to many other secondary complications (e.g., UTI, pressure sores), this article will focus on infectious respiratory conditions that are common with SCI.
For more information, refer to our articles on UTIs and Pressure Injuries!
Respiratory infections can occur in anyone, but people with SCI are at a higher risk for the following reasons:
The heart (in the center) and lungs are complexly interconnected. The lungs help oxygenate the blood, which is pumped by the heart.7
Infectious respiratory diseases can target either the upper respiratory tract (i.e., the nose, mouth, and throat), or the lower respiratory tract (i.e., the voice box, windpipe, airways into the lungs, and the lungs). Oxygen is required for each organ to function properly. When a part of your respiratory system becomes infected, the amount of air you breathe in may be reduced. This reduces the amount of oxygen available for the body, and may quickly affect the function of the brain and other organs. Moreover, these infections can spread all over the body (sepsis). Once it spreads, it becomes more difficult to treat.
As an SCI can negatively affect respiratory and immune function, the rates of respiratory diseases, such as bacterial pneumonia and influenza, among individuals with SCI is high. In fact, respiratory diseases account for just over 80% of all deaths after SCI. In addition, respiratory conditions often present more severely in SCI. This was shown in a (weak evidence) study, where people with SCI who contracted influenza or pneumonia were 37 times more likely to die from the infection compared to an able-bodied population. Two of the most common respiratory infections in people with SCI are pneumonia and the flu. These conditions are particularly infectious, and are caught through tiny droplets of fluid in the air that may be released as a cough or a sneeze. These droplets may fall on surfaces, and can spread if someone touches the surface, then touches their mouth or eyes.
Some (weak evidence) research done to predict who is more likely to experience respiratory illness after SCI indicated that those with a complete injury and those with tetraplegia are at an increased risk of dying from a respiratory related infection. Another study found that during acute care, those with a complete injury were at a greater risk of getting pneumonia. This is related to the lack of/weakened ability to cough and clear mucus from the airways. Secondary complications that may predispose people with SCI to respiratory illnesses include: obesity, heart disease, asthma, chronic obstructive pulmonary disease (COPD), chronic coughing, chronic existence of phlegm, wheezing, and the use of pulmonary medications.
Pneumonia is an infection caused by a bacteria or virus, which leads to an infection of the small air sacs in the lungs. It is one of the most common infections in acute SCI with about 30% of individuals with acute SCI experiencing pneumonia (weak evidence), dropping to about 3.5% in the chronic stages of SCI (i.e., 1-20 years post injury). Although the chance of getting pneumonia decreases after acute SCI, it is important to remember that pneumonia manifests more severely in people with SCI. That is to say, while your chances of getting pneumonia may be reduced at longer times after SCI, if you do get it, it is more severe.
Influenza, or the flu, is a respiratory condition caused by a virus. There are multiple types and sub-types of influenza, although type A and B are the strains that most often cause the flu season. The flu virus affects the nose, throat, and sometimes the lungs, and can lead to secondary conditions such as pneumonia. Flu vaccines are recommended, especially for people with SCI as they are a vulnerable population. There is weak evidence that supports the use of flu vaccines for people with SCI, as their immune system responds similarly to the vaccine compared to able-bodied individuals. That said, those with tetraplegia may have a reduced response to the vaccine. Animal studies suggest that vaccines may be less effective with higher levels of SCI. More research is required to determine the response to influenza vaccines after SCI.
There are many other infectious respiratory conditions that exist, such as the common cold, tuberculosis, and coronaviruses. However, little to no research has been done on the impact of these conditions in the SCI population. Although the available research is limited, it is important to note that individuals with SCI are still at an increased risk of contracting these conditions. The following section describes various respiratory infections in the able-bodied population unless otherwise noted.
Common Cold
The common cold (or simply, the cold) is a general term for a mild upper respiratory tract condition affecting the nose and throat. Common symptoms include stuffy nose, sneezing, sore throat, and cough. Unlike other conditions, there are multiple types of viruses that can cause a cold. Rhinoviruses, coronaviruses, and influenza viruses account for the majority of cases. The cold occurs most frequently in the fall, and decreases upon the arrival of spring. On average, a person will catch the cold once a year, but it is likely that this rate is underestimated.
Coronaviruses
A coronavirus (above), which gets its name from the spikes on the outside that resemble a crown, which is “corona” in Latin.10
Coronaviruses are responsible for many health conditions including the common cold, Middle East Respiratory Syndrome (MERS), Severe Acute Respiratory Syndrome (SARS), and novel coronavirus disease 2019 (COVID-19). These are the four strains of coronavirus now known to affect humans, and they are responsible for 10-30% of upper respiratory tract infections. Although these viruses are genetically related, they cause very different conditions. The severity of the conditions they may cause varies from mild (such as the common cold, described above), to severe (such as MERS, SARS, and COVID-19). Though MERS, SARS, and COVID-19 can all lead to pneumonia, each condition affects the body differently: MERS has a greater impact on the digestive system and kidneys, while SARS and COVID-19 most heavily impact the respiratory system, clotting function, and heart activity.
The 2019 Novel Coronavirus (COVID-19)
To date, few weak evidence studies on COVID-19 and SCI have been completed. This biggest take away is that the typical symptoms of COVID-19 as reported by the World Health Organization (i.e., cough, fever, and shortness of breath) are not necessarily applicable to people with spinal cord injuries. As coughing is often impaired with SCI, it can be absent in reported cases of COVID-19 in people with SCI. Instead, common symptoms of COVID-19 in people with SCI include a fever and feeling weaker than normal. Other symptoms that have been reported include shortness of breath, body aches/worsening pain, sweating, chest pain, and increased spasticity, a worsened ability to clear secretions, and abnormally fast breathing. Although people with SCI may have fewer of the typical COVID-19 symptoms, one study has found that they are more likely to experience COVID-19 more severely compared to able-bodied individuals. |
Tuberculosis
Tuberculosis is an infection of the lungs that can be caused by Mycobacterium tuberculosis bacteria. The presence of tuberculosis is higher in developing countries in comparison to developed countries. This is related to factors such as lower rates of vaccination and higher rates of HIV (an immune compromising condition) in developing countries. Treating tuberculosis is particularly difficult, as many strains of the virus/bacteria are resistant to drugs.
Upper respiratory tract infections
Upper respiratory tract infections are a group of conditions that affect the nose and throat. Some conditions include pharyngitis (sore throat) and laryngitis (inflammation of the voice box; when you lose your voice). These infections are of particular note to those using ventilators, as over 90% of pneumonia and hospitalizations start with an upper respiratory tract infection.
In order to avoid getting infectious respiratory conditions, prevention is key, especially in the community. Here is what you can do to stay healthy:
After an SCI, respiratory functions (i.e., breathing and coughing) and the immune system are compromised. Researchers are still unsure about why the immune system is suppressed. While there is some weak evidence for why the immune system changes after SCI, more clinical trials are required to determine the specific effects of SCI on the immune system.
Given the changes to respiratory and immune functioning after SCI, there is a higher risk of getting an infectious respiratory disease. The best thing to do is to work at prevention, which can be done through a variety of ways such as getting vaccinated and staying hydrated. Discuss all treatment options with your health providers to find out which treatments are suitable for you.
For a review of how we assess evidence at SCIRE Community and advice on making decisions, please see SCIRE Community Evidence.
SCIRE Community. “Respiratory Changes After SCI”. Available from: https://community.scireproject.com/topic/respiratory-changes/
SCIRE Community. “COVID-19 & SCI Infographic”. Available from: https://community.scireproject.com/covid-19/infographics/
Sheel AW, Welch JF, Townson AF (2018). Respiratory Management Following Spinal Cord Injury. In: Eng JJ, Teasell RW, Miller WC, Wolfe DL, Townson AF, Hsieh JTC, Connolly SJ, Noonan VK, Loh E, Sproule S, McIntyre A, Querée M, editors. Spinal Cord Injury Rehabilitation Evidence. Version 6.0. Vancouver: p. 1-72.
Available from: https://scireproject.com/evidence/respiratory-management-rehab-phase/
Tortora, G.J., and Derrickson .B.(2013).The Lymphatic System and Immunity. In Roesch, B. (Eds.), Principles of Anatomy and Physiology (pp. 366-447). Biologtical Science Textbooks
Allison, D. J., & Ditor, D. S. (2015). Immune dysfunction and chronic inflammation following spinal cord injury. Spinal Cord, 53(1), 14–18. https://doi.org/10.1038/sc.2014.184
Campagnolo, D. I., Dixon, D., Schwartz, J., Bartlett, J. A., & Keller, S. E. (2008). Altered innate immunity following spinal cord injury. Spinal Cord, 46(7), 477–481. https://doi.org/10.1038/sc.2008.4
Popovich, P., & McTigue, D. (2009). Beware the immune system in spinal cord injury. Nature Medicine, 15(7), 736–737. https://doi.org/10.1038/nm0709-736
Schwab, J. M., Zhang, Y., Kopp, M. A., Brommer, B., & Popovich, P. G. (2014). The paradox of chronic neuroinflammation, systemic immune suppression, autoimmunity after traumatic chronic spinal cord injury. Experimental Neurology, 258, 121–129. https://doi.org/10.1016/j.expneurol.2014.04.023
Riegger, T., Conrad, S., Schluesener, H. J., Kaps, H. P., Badke, A., Baron, C., … Schwab, J. M. (2009). Immune depression syndrome following human spinal cord injury (SCI): A pilot study. Neuroscience, 158(3), 1194–1199. https://doi.org/10.1016/j.neuroscience.2008.08.021
Herman, P., Stein, A., Gibbs, K., Korsunsky, I., Gregersen, P., & Bloom, O. (2018). Persons with Chronic Spinal Cord Injury Have Decreased Natural Killer Cell and Increased Toll-Like Receptor/Inflammatory Gene Expression. Journal of Neurotrauma, 35(15), 1819–1829. https://doi.org/10.1089/neu.2017.5519
Brommer, B., Engel, O., Kopp, M. A., Watzlawick, R., Müller, S., Prüss, H., … Schwab, J. M. (2016). Spinal cord injury-induced immune deficiency syndrome enhances infection susceptibility dependent on lesion level. Brain, 139(3), 692–707. https://doi.org/10.1093/brain/awv375
Burns, S. P. (2007). Acute Respiratory Infections in Persons with Spinal Cord Injury. Physical Medicine and Rehabilitation Clinics of North America, 18(2), 203–216. https://doi.org/10.1016/j.pmr.2007.02.001
Northwest Regional Spinal Cord Injury System. (2004, October 12). Common respiratory problems in SCI – What you need to know. http://sci.washington.edu/info/forums/reports/common_respiratory.asp
DeVivo, M. J., Black, K. J., & Stover, S. L. (1993). Causes of death during the first 12 years after spinal cord injury. Archives of Physical Medicine and Rehabilitation, 74(3), 248–254. https://doi.org/10.5555/uri:pii:000399939390132T
Stolzmann, K. L., Gagnon, D. R., Brown, R., Tun, C. G., & Garshick, E. (2010). Risk factors for chest illness in chronic spinal cord injury: a prospective study. American Journal of Physical Medicine & Rehabilitation, 89(7), 576–583. https://doi.org/10.1097/PHM.0b013e3181ddca8e
Berlly, M., & Shem, K. (2007). Respiratory management during the first five days after spinal cord injury. Journal of Spinal Cord Medicine, 30(4), 309–318. https://doi.org/10.1080/10790268.2007.11753946
McKinley, W. O., Jackson, A. B., Cardenas, D. D., & DeVivo, M. J. (1999). Long-term medical complications after traumatic spinal cord injury: A Regional Model Systems Analysis. Archives of Physical Medicine and Rehabilitation, 80(11), 1402–1410. https://doi.org/10.1016/S0003-9993(99)90251-4
Trautner, B. W., Atmar, R. L., Hulstrom, A., & Daroiuche, R. O. (2004). Inactivated Influenza Vaccination for People With Spinal Cord Injury. Arch Phys Med Rehabil, 85(11), 1886–1889. https://doi.org/10.1038/jid.2014.371
Centers for Disease Control and Prevention. (2020a). Understanding influenza viruses. https://www.cdc.gov/flu/about/viruses/index.htm
Centers for Disease Control and Prevention. (2020b). Prevent seasonal flu. https://www.cdc.gov/flu/prevent/index.html
Burns, S. P. (2007). Acute Respiratory Infections in Persons with Spinal Cord Injury. Physical Medicine and Rehabilitation Clinics of North America, 18(2), 203–216. https://doi.org/10.1016/j.pmr.2007.02.001
Rao, N. M. (2015). Swine flu the pandemic disease – preventive measures to take. Journal of Medical Science and Technology, 4(1), 1–2.
Heikkinen, T., & Järvinen, A. (2003). The common cold. Lancet, 361(9351), 51–59. https://doi.org/10.1016/S0140-6736(03)12162-9
Paules, Catharine, I., Marston, H. D., & Fauci, A. S. (2020). Coronavirus Infections – More than Just the Common Cold. Journal of the American Medical Association, 323(8), 707–708. https://doi.org/10.1007/82
Stillman, M. D., Capron, M., Alexander, M., Di Giusto, M. L., & Scivoletto, G. (2020). COVID-19 and spinal cord injury and disease: results of an international survey. Spinal Cord Series and Cases, 6(1), 21.
Rodriguez-Cola, M., Jimenez-Velasco, I., Henares-Gutierrez, F., Lopez-Dolado, E., Gambarrutta-Malfatti, C., Vargas-Baquero, E., & Gil-Agudo, A. (2020). Clinical features of coronavirus disease 2019 (COVID-19) in a cohort of patients with disability due to spinal cord injury.
Righi, G., Del, G., & Popolo, G. Del. (2020). COVID-19 tsunami : the first case of a spinal cord injury patient in Italy. Spinal Cord Series and Cases, 3–7. https://doi.org/10.1038/s41394-020-0274-9
Mayo Clinic (2020). Tuberculosis. https://www.mayoclinic.org/diseases-conditions/tuberculosis/symptoms-causes/syc-20351250
World Health Organization. (2020). Tuberculosis. https://www.who.int/news-room/fact-sheets/detail/tuberculosis
LaVela, S. L., Smith, B., & Weaver, F. M. (2007). Perceived Risk for Influenza in Veterans With Spinal Cord Injuries and Disorders. Rehabilitation Psychology, 52(4), 458–462. https://doi.org/10.1037/0090-5550.52.4.458
Ronca, E., Miller, M., Brinkhof, M. W. G., Jordan, X., Léger, B., Baumberger, M., … Fekete, C. (2020). Poor adherence to influenza vaccination guidelines in spinal cord injury: results from a community-based survey in Switzerland. Spinal Cord, 58(1), 18–24. https://doi.org/10.1038/s41393-019-0333-x
Weaver, F. M., Smith, B., LaVela, S., Wallace, C., Evans, C. T., Hammond, M., & Goldstein, B. (2007). Interventions to increase influenza vaccination rates in veterans with spinal cord injuries and disorders. Journal of Spinal Cord Medicine, 30(1), 10–19. https://doi.org/10.1080/10790268.2007.11753908
Author: Sharon Jang | Reviewer: Viet Vu | Published: 10 March 2020 | Updated: ~
Sleep disordered breathing is common after a spinal cord injury (SCI). This page explains what sleep disordered breathing is, why it occurs, what factors influence it, and current management options.
Key Points
Obstructive sleep apnea occurs when the throat muscles relax (highlighted by the red circle), resulting in a blockage of your airway.1
Sleep disordered breathing is an umbrella term for conditions that involve an interruption of breathing throughout the night. In research, sleep disordered breathing is evaluated by observing two key factors:
When you sleep, the body normally goes into a state of hypoventilation, or a slow and shallow breathing. This results in a decrease in oxygen circulating in the blood. However, weak evidence suggests that after an SCI, hypoventilation becomes more prevalent during sleep when compared to able-bodied people. Among those with SCI, hypoventilation occurs more often in individuals with tetraplegia versus paraplegia.
The two most common disorders under sleep disordered breathing include:
Of the two types of sleep apneas, obstructive sleep apnea is more prevalent among individuals with SCI of all levels. However, central sleep apnea is more prevalent among individuals with cervical level injuries. Narcotic use can also increase the risk of central sleep apnea. It is important to note that some patients have mixed sleep apnea, a combination of obstructive and central sleep apnea.
The prevalence of sleep apnea in individuals with spinal cord injury is two to five times greater than that in the non-SCI population. In the SCI population, research has found that sleep apnea rates vary from 27-82%. The variation in prevalence can be attributed to different diagnostic measures used in research studies (e.g., evaluating sleep apnea in a lab versus home setting) and the way each study defines sleep apnea. Level of injury and type of injury also influence the prevalence of sleep apnea in the SCI population.
A few hypotheses have been made by scientists as to why sleep apnea is prevalent in SCI. In general, sleep apnea is attributed to a complex interaction of a variety of factors:
Sleep disordered breathing is more prevalent in individuals with tetraplegia compared to those with paraplegia. Having a higher level of injury is usually associated with decreased muscle functioning and neural control over your organs. These impairments can create troubles with breathing, specifically with inhaling, exhaling, and the amount of air your lung can hold.
Refer to our article on Respiratory Changes After SCI for more information.
After an SCI, your body becomes more sensitive to the amount of carbon dioxide circulating throughout your body. So, when there is a slight increase in carbon dioxide in the body, the brain senses it as a large change, which cues the body to hyperventilate, or rapidly breathe. However, since the change in carbon dioxide was small to start with, hyperventilation results in excess removal of carbon dioxide, resulting in very low carbon dioxide levels. During sleep, breathing is dependent on the amount of carbon dioxide circulating in the body. If this level drops below the level required for breathing, then central sleep apnea occurs. While some researchers believe this may be a cause of central sleep apnea, others note that there is currently only weak evidence to support this hypothesis.
Measures of body composition, including body mass index (BMI), neck circumference, and waist circumference, may be linked to the prevalence of sleep disordered breathing. Weak research evidence has suggested that a greater neck circumference or BMI can increase the odds of having sleep disordered breath. This is concerning for individuals with SCI, as 44-66% of this population are overweight or obese. However, other studies have found no relationship between BMI or neck circumference and sleep disordered breathing in SCI.
One weak evidence study has suggested that there is more than a 50% increase in apneic events that occur when you sleep on your stomach or on your back, rather than on your side. More specifically, tetraplegics who sleep on their backs experienced more apneas and hypopneas per hour compared to those who slept in other positions. Despite this evidence, other researchers found that sleeping on your back enhances overall breathing functions after SCI. More research is required to determine the optimal sleeping position for health benefits, keeping in mind that bed mobility and turns overnight also help to maintain skin health.
Common medications used by individuals with SCI can also impact breathing during sleep. These include: narcotics, baclofen, benzodiazepines (lorazepam, diazepam, clonazepam), testosterone, and heart medications to treat high blood pressure or arrhythmias. Although medications can cause sleep disordered breathing, they are not likely to be the main contributor to the issue.
Individuals with tetraplegia may find it harder to breathe in with their nose (i.e., nasal resistance) because swelling of the blood vessels and a thickening of the mucus in the nose is a common effect of cervical spinal cord injury. One moderate evidence study found that individuals with tetraplegia experienced greater nasal resistance in comparison with able-bodied individuals. Since increases in breathing resistance can cause the airways to collapse, some researchers believe that this may contribute to the higher prevalence of obstructive sleep apnea in individuals with tetraplegia.
Some of the most common symptoms of sleep apnea in individuals with spinal cord injury include:
Although the above are symptoms of sleep apnea, it is important to note that these same symptoms can result from secondary complications from a spinal cord injury (e.g., pain, spasticity, posture).
CPAP and BiPAP are powered by a machine which regulates the flow of air. These machines are connected via a tube to a mask worn over the nose and mouth..9
The first line of treatment for sleep disordered breathing generally includes lifestyle changes consisting of weight loss and the avoidance of alcohol and smoking. These lifestyle changes are normally done in conjunction with the use of a continuous positive airway pressure (CPAP) machine. CPAP machines act as a “pneumatic splint” that holds the airway open using a continuous pressure of air. To use a CPAP machine, a mask or nasal pillows are worn over the face/nose overnight.
CPAP machines are commonly used to address sleep apnea, and their effectiveness for the SCI population is supported by some weak evidence research studies. Although CPAP machines can help with breathing, multiple weak evidence research articles report poor adherence in using CPAP machines. Some of these reasons include:
Although CPAP machines have the potential to help with sleep disordered breathing, more research is required to determine how helpful CPAP machines are to individuals with SCI, and how we can improve adherence to this treatment.
Bilevel positive airway pressure (BiPAP) machines operate similarly to CPAP machines in that air pressure is acts as a “pneumatic splint”. however, BiPAP machines do not deliver a constant pressure of air. Exhaling when using a CPAP machine may be difficult, as breathing against an inflow of air requires effort. The BiPAP machine is unique as the pressures it exerts varies with inhalation/exhalation. Normally, the BiPAP machine will be set to a higher pressure for inhalation, and to a reduced pressure during exhalation to facilitate this process. While there have been some thoughts that BiPAP machines may benefit the able-bodied population, more research is required to determine the effectiveness of BiPAP machines in an SCI population. It is worth a discussion with your doctor if you are not tolerating a CPAP machine to trial a BiPAP machine to treat sleep apnea.
An example of a dental appliance. A gap exists between the teeth to help promote airflow when sleeping.10
Dental appliances are sometimes an alternative to CPAP if an individual exhibits mild sleep disordered breathing. Dental appliances fit in the mouth like a mouth guard, and help pull the jaw and the tongue forward to prevent obstructive sleep apnea by opening up the airway. Although there has been a lot of literature supporting the use of dental appliances in an able-bodied population, more research needs to be conducted in an SCI population.
In the UVPPP surgical procedure, extra tissue is removed from the roof of your mouth and/or from your throat.11
Surgical interventions for sleep disordered breathing are often the last resort, after CPAP or BiPAP fail to work. There are a variety of surgical procedures that are used to aid obstructive sleep apnea, many of which involve reducing or repositioning the soft tissue at the back of the throat. One of the most common surgical procedures is an uvulopalatopharyngoplasty (UVPPP), whereby the soft tissues at the back of your mouth and throat are reduced and removed. However, the success rate for this procedure is variable, and there is only weak evidence to support this technique in able-bodied populations. Moreover, sleep disordered breathing in SCI may result from complex interactions between a variety of factors including level of injury, weight, sleep position, and medications. Although obstructive sleep apnea is common in SCI, researchers are unsure whether it stems from the structure of the throat or changes accompanied by an SCI. The evidence for surgical procedures to aid obstructive sleep apnea after SCI is sparse and requires more research.
Sleep disordered breathing, or a lack of breathing during sleep, is two to five times more prevalent in the SCI population compared to the able-bodied population. This increase in prevalence is believed to be related to a variety of factors including weight, level of injury, sleep position, and medications. While there are a variety of non-invasive and invasive procedures to manage sleep disordered breathing, more research is required to determine which treatment is most effective in an SCI population.
For a review of how we assess evidence at SCIRE Community and advice on making decisions, please see SCIRE Community Evidence.
Sheel AW, Welch J, Townson AF (2018). Respiratory Management Following Spinal Cord Injury. In: Eng JJ, Teasell RW, Miller WC, Wolfe DL, Townson AF, Hsieh JTC, Connolly SJ, Noonan VK, Loh E, Sproule S, Querée M, McIntyre A, editors. Spinal Cord Injury Rehabilitation Evidence. Version 6.0. Vancouver: p. 1-72.
Available from: https://scireproject.com/evidence/rehabilitation-evidence/respiratory-management/
Bascom, A. T., Sankari, A., Goshgarian, H. G., & Badr, M. S. (2015). Sleep onset hypoventilation in chronic spinal cord injury. Physiological Reports, 3(8), 1–10.
Castriotta, R. J., & Murthy, J. N. (2009). Hypoventilation after spinal cord injury. Seminars in Respiratory and Critical Care Medicine, 30(3), 330–338.
Chiodo, A. E., Sitrin, R. G., & Bauman, K. A. (2016). Sleep disordered breathing in spinal cord injury: A systematic review. Journal of Spinal Cord Medicine, 39(4), 374–382.
Fuller, D. ., Lee, K., & Tester, N. J. (2014). The impact of spinal cord injury on breathing during sleep, 27(4), 1–19.
Sankari, A., Vaughan, S., Bascom, A., Martin, J. L., & Badr, M. S. (2019). Sleep-Disordered Breathing and Spinal Cord Injury: A State-of-the-Art Review. Chest, 155(2), 438–445. Retrieved from https://doi.org/10.1016/j.chest.2018.10.002
Baydur A, Adkins RH, Milic-Emili J. (2001). Lung mechanics in individuals with spinal cord injury: effects of injury level and posture. Journal of applied physiology, 90, 405–411.
Berlowitz, D. J., Brown, D. J., Campbell, D. A., & Pierce, R. J. (2005). A longitudinal evaluation of sleep and breathing in the first year after cervical spinal cord injury. Archives of Physical Medicine and Rehabilitation, 86(6), 1193–1199.
Chiodo, A. E., Sitrin, R. G., & Bauman, K. A. (2016). Sleep disordered breathing in spinal cord injury: A systematic review. Journal of Spinal Cord Medicine, 39(4), 374–382.
Mason, M., Cj, C., & Smith, I. (2015). Effects of opioid, hypnotic and sedatingmedications on sleep- disordered breathing in adults with obstructive sleep apnoea (Review). Cochrane Database of Systematic Reviews, (7).
Fuller, D. ., Lee, K., & Tester, N. J. (2014). The impact of spinal cord injury on breathing during sleep, 27(4), 1–19.
McEvoy, R. D., Mykytyn, I., Sajkov, D., Flavell, H., Marshall, R., Antic, R., & Thornton, A. T. (1995). Sleep apnoea in patients with quadriplegia. Thorax, 50(6), 613–619.
Oksenberg, A., Silverberg, D. S., Arons, E., & Radwan, H. (1997). Positional vs nonpositional obstructive sleep apnea patients: Anthropomorphic, nocturnal polysomnographic, and multiple sleep latency test data. Chest, 112(3), 629–639.
Sankari, A., Vaughan, S., Bascom, A., Martin, J. L., & Badr, M. S. (2019). Sleep-Disordered Breathing and Spinal Cord Injury: A State-of-the-Art Review. Chest, 155(2), 438–445. Retrieved from https://doi.org/10.1016/j.chest.2018.10.002
Wijesuriya, N. S., Lewis, C., Butler, J. E., Lee, B. B., Jordan, A. S., Berlowitz, D. J., & Eckert, D. J. (2017). High nasal resistance is stable over time but poorly perceived in people with tetraplegia and obstructive sleep apnoea. Respiratory Physiology and Neurobiology, 235, 27–33. Retrieved from http://dx.doi.org/10.1016/j.resp.2016.09.014
Chiodo, A. E., Sitrin, R. G., & Bauman, K. A. (2016). Sleep disordered breathing in spinal cord injury: A systematic review. Journal of Spinal Cord Medicine, 39(4), 374–382.
Fuller, D. ., Lee, K., & Tester, N. J. (2014). The impact of spinal cord injury on breathing during sleep, 27(4), 1–19.
Burns, S. P., Little, J. W., Hussey, J. D., Lyman, P., & Lakshminarayanan, S. (2000). Sleep apnea syndrome in chronic spinal cord injury: Associated factors and treatment. Archives of Physical Medicine and Rehabilitation, 81(10), 1334–1339.
Burns, S. P., Rad, M. Y., Bryant, S., & Kapur, V. (2005). Long-term treatment of sleep apnea in persons with spinal cord injury. American Journal of Physical Medicine and Rehabilitation, 84(8), 620–626.
Fuller, D. ., Lee, K., & Tester, N. J. (2014). The impact of spinal cord injury on breathing during sleep, 27(4), 1–19.
Rotenberg, B. W., Vicini, C., Pang, E. B., & Pang, K. P. (2016). Reconsidering first-line treatment for obstructive sleep apnea: A systematic review of the literature. Journal of Otolaryngology – Head and Neck Surgery, 45(1), 1–9. Retrieved from http://dx.doi.org/10.1186/s40463-016-0136-4
Stockhammer, E., Tobon, A., Michel, F., Eser, P., Scheuler, W., Bauer, W., … Zach, G. A. (2002). Characteristics of sleep apnea syndrome in tetraplegic patients. Spinal Cord, 40, 286–294.
Tromans, A. M., Mecci, M., Barrett, F. H., Ward, T. A., & Grundy, D. J. (1998). The use of the BiPAP® biphasic positive airway pressure system in acute spinal cord injury. Spinal Cord, 36(7), 481–484.
Author: Sharon Jang | Reviewer: Cathy Nevens | Published: 7 September 2019 | Updated: ~
Abdominal binders are simple pieces of equipment that are used to support the abdomen. This page outlines what abdominal binders are and how they are used after spinal cord injury (SCI).
Key Points
Abdominal binders wrap around to support the abdomen when the abdominal muscles are weak or paralyzed. They are normally worn under the shirt.1
An abdominal binder is an elastic piece of material that is placed normally placed around the lower torso to apply pressure to the abdomen. Abdominal binders should fit snugly around the torso and be tight enough to provide support, but should not be uncomfortable. Abdominal binders are typically worn under the shirt and are mainly used to improve circulation and breathing when in an upright position. They are also sometimes used to help maintain balance and stability of the trunk and to support sagging of the abdomen (sometimes called “quad belly”) that can happen when the abdominal muscles are weak.
There are two main types of abdominal binders: elastic and non-elastic binders. Each of these types will have a number of different models or designs available that may be used. Talk to your health providers about what type are most appropriate for you.
The most common type of abdominal binder used by people with SCI is made from a stretchy elastic fabric that is placed around the abdomen and closed with Velcro. The material mimics the nature of the abdominal muscles by providing some pressure but also allowing the abdomen to expand and recoil while breathing. Some elastic abdominal binders have additional supports built into them that may be used to assist with balance and stability.
Non-elastic abdominal binders include pieces of equipment such as a corset, girdle, straps or mechanical device to support the abdomen. These are made from a non-stretchy material that provides greater support. Non-elastic abdominal binders are not used as often after SCI because they have greater potential to injure the skin and may also restrict the abdomen while breathing, which may contribute to an abnormal breathing pattern.
People with cervical and thoracic SCI may experience breathing problems because of a loss of nerve control to the diaphragm and other breathing muscles (including the abdominal muscles). This causes the diaphragm to sit too low in the abdomen so it cannot work optimally.
Abdominal binders are thought to mimic some of the function of the abdominal muscles to help support breathing. The binder compresses the abdomen, which increases pressure and may help to raise the diaphragm into a better position for breathing.
There is evidence that abdominal binding in people with tetraplegia can improve respiratory function. Studies have shown that the use of abdominal binders can improve an individual’s ability to inhale and exhale. Overtime, the use of an abdominal binder can strengthen the muscles that are used to inhale. The design of the abdominal binder may also influence its effectiveness. For example, one (weak evidence) study found that a custom girdle may cause individuals to perceive breathing as easier.
More research is required to find out how using an abdominal binder strengthens the diaphragm and whether this leads to easier breathing. Abdominal binding for people with SCI should be introduced gradually due to potential adverse effects on one’s ability to breathe.
Refer to our article on Respiratory Changes After SCI for more information!
Many people with SCI experience a drop in blood pressure when moving from a lying or sitting position to an upright position. This is known as orthostatic hypotension. This condition happens because a loss of nerve function can impair the body’s ability to tighten (constrict) the blood vessels and change heart rate, which is an important part of maintaining blood pressure in different positions.
Because abdominal binders wrap around and compress the abdomen, they may help to increase pressure in the abdominal area. This may help to prevent blood pooling in the blood vessels in the abdomen when upright, which may help maintain blood pressure and allow better circulation.
There is conflicting evidence based on limited research that abdominal binders have any effect on cardiovascular responses in people with SCI. One study found that abdominal binders do not have any effect on average blood pressure or other cardiovascular responses. However, other studies suggest that abdominal binders in combination with leg stockings may have an effect on cardiovascular responses during lower intensity arm exercise.
Refer to our article on Orthostatic Hypotension for more information!
There is some weak evidence for the use of an abdominal binder to improve speech. One study found that participants with difficulty speaking due to cervical level injuries were able to produce more natural sounding speech, were able to speak louder, and improved overall voice quality with the use of an abdominal binder. Meanwhile, another study has shown that using an abdominal binder can extend the length that sound is produced for.
Abdominal binders may be used to support the abdomen to reduce the appearance of “quad belly”.5
Some individuals use an abdominal binder as they perceive it helps to support their trunk or assist with sitting balance. In addition, some individuals find that it helps them balance when performing two-handed exercises. However, no research has been done to support this. Some individuals may use a binder to reduce the appearance of the abdomen sagging forward, sometimes called “quad belly”.
Abdominal binders are considered safe for most people. In all cases, the skin under the binder should be regularly checked for pressure sores. However, there are situations in which abdominal binders may not be appropriate and carry possible risks. Please consult a health provider for detailed safety information.
Refer to our articles on Pressure Injuries, Spasticity, and Autonomic Dysreflexia for more information!
Abdominal binders are a physical treatment that supports the abdomen when the abdominal muscles are weak or paralyzed.
The support from the binder can improve cardiovascular and respiratory responses including blood pressure and breathing.
Some individuals may use a binder to help with balance and stabilizing the trunk but there is no research evidence to support this.
Some individuals may use a binder to reduce the appearance of abdominal muscles that bulge out following paralysis.
For a review of how we assess evidence at SCIRE Community and advice on making decisions, please see SCIRE Community Evidence.
This page has been adapted from SCIRE Professional “Respiratory Management” and “Orthostatic Hypotension” Modules:
Sheel AW, Reid WD, Townson AF (2018). Respiratory Management Following Spinal Cord Injury. In: Eng JJ, Teasell RW, Miller WC, Wolfe DL, Townson AF, Hsieh JTC, Connolly SJ, Noonan VK, Loh E, Sproule S, Querée M, McIntyre A, editors. Spinal Cord Injury Rehabilitation Evidence. Version 6.0. Vancouver: p. 1-72.
Available from: scireproject.com/evidence/respiratory-management/
Krassioukov A, Wecht JM, Teasell RW, Eng JJ (2014). Orthostatic Hypotension Following Spinal Cord Injury. In Eng JJ, Teasell RW, Miller WC, Wolfe DL, Townson AF, Hsieh JTC, Connolly SJ, Noonan VK, Loh E, McIntyre A, editors. Spinal Cord Injury Rehabilitation Evidence. Version 5.0. Vancouver: p 1- 26.
Available from: scireproject.com/evidence/orthostatic-hypotension/non-pharmacological-management-of-oh/
Cornwell, P., Ward, E., Lim, Y., & Wadsworth, B. (2014). Impact of an Abdominal Binder on Speech Outcomes in People With Tetraplegic Spinal Cord Injury: Perceptual and Acoustic Measures. Topics in Spinal Cord Injury Rehabilitation, 20(1), 48–57. https://doi.org/10.1310/sci2001-48
Wadsworth, B. M., Haines, T. P., Cornwell, P. L., Rodwell, L. T., & Paratz, J. D. (2012). Abdominal Binder Improves Lung Volumes and Voice in People With Tetraplegic Spinal Cord Injury. YAPMR, 93(12), 2189–2197. https://doi.org/10.1016/j.apmr.2012.06.010
Author: SCIRE Community Team | Reviewer: Shannon Sproule | Published: 21 November 2017 | Updated: ~
Inspiratory muscle training is a treatment used to help with breathing and coughing after spinal cord injury (SCI). This page introduces inspiratory muscle training and its use after SCI.
Key Points
Inspiratory muscle training describes a number of different techniques in which simple equipment is used to strengthen the muscles used to breathe in (inspire). The equipment is used to make breathing in more challenging. This causes the breathing muscles to work harder, so that they can adapt and become stronger with training.
Speak with a health provider before using inspiratory muscle training to make sure it is safe and suitable for you and to learn how to use the equipment correctly.
Inspiratory muscle training is done using a device that makes breathing in more challenging. The device has some type of mechanism that creates resistance when the person breathes in, but allows breathing out to occur freely. There are several different types of inspiratory muscle training devices:
Different types of inspiratory muscle trainers – Top: threshold trainer; bottom: resistive trainer.2
Your health provider will assist you with selecting an appropriate trainer and supervise your early training. The device is held to the mouth with a clip over the nose. Some people with reduced hand movement will need someone to position and hold the trainer during each training session. Alternatively, tilting the chair backwards or using a stand to hold the trainer may assist with positioning the mouthpiece independently.
Inspiratory muscle training involves regular sessions of breathing exercises consisting of a set number of breaths and sets. Sessions often last around 30 minutes and are done 2 to 3 times per day. However, the ideal protocol for inspiratory muscle training for people with SCI is not yet known.
Inspiratory muscle training is usually done for at least 6 weeks to allow enough time for the muscles to become stronger and adapt to training. Like other exercise, training should be continued regularly for strength to be maintained.
Cervical and thoracic spinal cord injuries can cause paralysis or weakness of muscles of the neck, chest, and abdomen that are important for breathing and coughing. This can cause breathing problems that can contribute to shortness of breath, fatigue, and a need for mechanical ventilation.
Depending on the level of injury of the SCI, some of the muscles of breathing may still be able to be controlled. In some cases, strengthening these muscles can help to compensate for weakness in other muscles and improve breathing ability.
Inspiratory muscle training is used to strengthen the muscles that can be controlled to improve independent breathing ability. It may also be used to help prevent medical complications related to breathing and coughing problems, such as lung infections like pneumonia.
Every person is unique, and how much inspiratory muscle training will benefit any individual depends on the characteristics of their SCI and their unique life circumstances. That being said, in general, inspiratory muscle training is considered most beneficial for people with injuries in the mid-cervical to mid-thoracic spinal cord. These types of injuries tend to have breathing problems that could benefit from inspiratory muscle training and also have muscles available to train. People with upper cervical SCI usually have greater impairment of the breathing muscles that often requires more extensive breathing support instead, such as ongoing mechanical ventilation.
There are certain situations where extra attention is needed to determine whether inspiratory muscle training is appropriate and safe. Consult a qualified health provider for further safety information.
Restrictions of precautions for using inspiratory muscle training may include:
Inspiratory muscle training is generally safe when used appropriately (see above for a list of situations in which inspiratory muscle training may not be safe). However, it is important to seek advice from a health provider to determine if this treatment is the best option for you.
Some possible risks and side effects of using inspiratory muscle training may include:
Several research studies have shown that inspiratory muscle training helps to improve breathing after spinal cord injury. Inspiratory muscle training was found to improve the strength and endurance of the breathing muscles. As well, it may reduce shortness of breath and chest infections in some people with SCI. These findings are supported by moderate evidence from five studies and weak evidence from six studies.
Research on whether inspiratory muscle training is effective in helping with speaking and coughing and what its long-term effects are is currently lacking.
Breathing difficulties are common among people with thoracic and cervical injuries. Inspiratory muscle training is a safe treatment option for increasing strength of breathing muscles to aid breathing. The research evidence suggests inspiratory muscle training is effective in increasing strength and endurance of the breathing muscles after SCI. It is important to discuss treatment options with your health providers to find out which treatments are suitable for you.
For a review of how we assess evidence at SCIRE Community and advice on making decisions, please see SCIRE Community Evidence.
Parts of this page have been adapted from the SCIRE Project (Professional) “Respiratory Management” Chapter:
Sheel AW, Reid WD, Townson AF, Ayas N (2014). Respiratory Management Following Spinal Cord Injury. In: Eng JJ, Teasell RW, Miller WC, Wolfe DL, Townson AF, Hsieh JTC, Connolly SJ, Noonan VK, Loh E, McIntyre A, editors.
Spinal Cord Injury Rehabilitation Evidence. Version 5.0. Vancouver: p. 1-54. Available from: https://scireproject.com/evidence/rehabilitation-evidence/respiratory-management/
Evidence for “How effective is inspiratory muscle training for people with SCI?” is based on the following studies:
[1] Van Houtte S, Vanlandewijck Y, Kiekens C, Spengler CM, Gosselink R. Patients with acute spinal cord injury benefit from normocapnic hyperpnoea training. J Rehabil Med 2008; 40: 119-125.
[2] Mueller G, Hopman MTE, and Perret C. Comparison of respiratory muscle training methods in individuals with motor complete tetraplegia. Top Spinal Cord Inj Rehabil 2012; 18(2): 118-121.
[3] Mueller G, Hopman MTE, and Perret C. Comparison of respiratory muscle training methods in individuals with motor and sensory complete tetraplegia: a randomized controlled trial. J Rehabil Med 2013; 45: 248-253.
[4] Loveridge B, Badour M, Dubo H. Ventilatory muscle endurance training in quadriplegia: effects on breathing pattern. Paraplegia 1989; 27: 329-339.
[5] Liaw M-Y, Lin M-C, Cheng P-T, Wong M-K A, Tang F-T. Resistive inspiratory muscle training: its effectiveness in patients with acute complete cervical injury. Arch Phys Med Rehabil 2000; 81: 752-756.
[6] Derrickson J, Ciesla N, Simpson N, Imle PC. A comparison of two breathing exercise programs for patients with quadriplegia. Physical Therapy 1992; 72: 763-769.
[7] Tamplin J, Baker FA, Grocke D, Brazzale DJ, Pretto JJ, Ruehland WR, Buttifant M, Brown DJ and Berlowitz DJ. Effect of singing on respiratory function, voice and mood after quadriplegia: a randomized controlled trial. Arch Phys Med Rehabil 2013; 94: 426-434.
[8] Litchke LG, Lloyd LK, Schmidt EA, Russian CJ, and Reardon RF. Effects of concurrent respiratory resistance training on health-related quality of life in wheelchair rugby athletes: a pilot study. Top Spinal Cord Inj Rehabil 2012; 18(3): 264-272.
[9] Ehrlich M, Manns PJ, Poulin C. Respiratory training for a person with C3-C4 tetraplegia. Aust J Physiother 1999; 45(4): 301-307.
[10] Uijl SG, Houtman S, Folgering HT, Hopman MT. Training of the respiratory muscles in individuals with tetraplegia. Spinal Cord 1999; 37: 575-9.
[11] Rutchik A, Weissman AR, Almenoff PL, Spungen AM, Bauman WA, Grimm DR. Resistive IMT in subjects with chronic spinal cord injury. Arch Phys Med Rehabil 1998; 79: 293-297.
[12] Hornstein S, Ledsome JR. Ventilatory muscle training in acute quadriplegia. Physiotherapy Canada 1986; 38: 145-149.
[13] Gross D, Ladd HW, Riley EJ, Macklem PT, Grassino A. The effect of training on strength and endurance of the diaphragm in quadriplegia. Am J Med 1980; 68: 27-35.
Other references:
Postma K, Haisma JA, Hopman M, Bergen MP, Stam HJ, Bussmann JB. Resistive inspiratory muscle training in people with spinal cord injury during inpatient rehabilitation: a randomized controlled trial. Phys Ther 2014; 94(12): 1709-1719.
McConnell A. Respiratory muscle training: theory and practice. London: Churchill Livingston; 2013. Chapter 6, Implementing respiratory muscle training; p.149-173.
Berlowitz DJ, Tamplin J. Respiratory muscle training for cervical spinal cord injury. Cochrane Database of Syst Rev 2013, 7: CD008507.
Spungen AM, Grimm DR, Lesser M, Bauman WA, Almenoff PL. Self-reported prevalence of pulmonary symptoms in subjects with spinal cord injury. Spinal Cord 1997; 35: 652-657,
Reid WD, Geddes EL, Brooks D, O’Brien K, Crowe J. Inspiratory muscle training in chronic obstructive pulmonary disease. Special Series on Skeletal Muscle Training. Physiotherapy Canada 2004; 56:128-142.
Geddes EL, Reid WD, Brooks D, Crowe J, O’Brien K. A Primer on Inspiratory Muscle Trainers. Buyers Guide for the European Respiratory Society 2006.
Geddes EL, Reid WD, Crowe J., O’Brien K, Brooks D. Inspiratory muscle training in adults with chronic obstructive pulmonary disease: A systematic review. Respir Med 2005; 99: 1440-1458.
Sheel AW, Reid WD, Townson AF, Ayas NT, Konnyu KJ. Effects of exercise training and inspiratory muscle training in spinal cord injury: a systematic review. J Spinal Cord Med 2008; 31(5): 500-8.
Image Credits