Author: Sharon Jang, Vanessa Mok | Reviewer: Peggy Assinck | Published: 13 May 2020 | Updated: ~
Since the discovery of stem cell therapy, researchers have attempted to determine whether it can be a safe and effective treatment for spinal cord injury (SCI). This page addresses what stem cell therapy is and its current place in SCI.
Key Points
- Stem cells are cells that have the ability to self-renew indefinitely and specialize into different types of functioning cells
- Stem cells treatment following SCI has been proposed to prevent further damage to the spinal cord, bridge lesion sites, and promote the regrowth of nerves
- There is limited evidence that stem cell therapy is safe and effective in humans (across different disorders) and therefore more work needs to be done to confirm safety and potential effectiveness
- There are currently no stem cell therapies approved for SCI. However, many clinics worldwide continue to offer cell transplantation-based treatments including stem cell therapies that have very little scientific evidence to back them up
- It is important to stay well-informed about ongoing research and importantly the potential risks involved in stem cell-based treatments
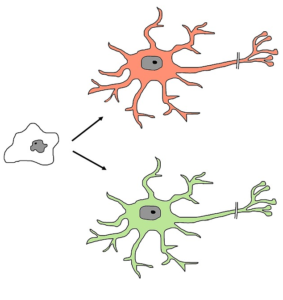
Stems cells have the ability to self-renew (blue arrow), and turn into different cell types such as muscle cells, skin cells, and nerve cells (pictured).1
All tissues and organs in the body originate from stem cells. Stem cells are unspecialized, blank cells that have the ability to turn into specialized cells (e.g., neural cells, muscle cells, etc) through chemical signals in the body. These cells are responsible for maintaining and repairing the tissue they are found in. Stem cells are defined by two characteristic features: 1) their ability to self-renew (i.e., make copies of themselves) and 2) their ability to differentiate into functioning cell types (i.e., they can turn into specialized cells). Some stem cells are only found in certain parts of the body or at certain stages of life. There are four main types of stem cells that are currently used in stem cell therapy or research.
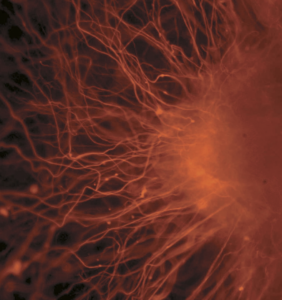
Neurons extending from a group of embryonic stem cells.2
Embryonic stem cells
Embryonic stem cells are sourced from embryos that are about a week old. In Canada, embryos cannot be specifically created for research purposes. Instead, these stem cells originate from unused embryos from fertility clinics. Their use in research raises ethical controversies as the embryos are destroyed during the harvesting process. These cells are pluripotent which means that they can turn into any cell in the body except for the umbilical cord and placenta.
Adult stem cells
Adult stem cells (also known as somatic stem cells or tissue-specific stem cells) are sourced from organ tissues (e.g., the heart, bone marrow, fat) found in the bodies of infants, children, and adults. They can also be produced from discarded placentas and umbilical cords. They have less capability to self-divide and specialize into different cell types compared to embryonic stem cells. Rather, adult stem cells more commonly produce tissue types in which they are found, so are multipotent. For instance, blood stem cells only have the potential to generate red blood cells, white blood cells, and platelets.
Induced pluripotent stem cells
Advancements in technology have allowed researchers to reprogram specialized adult cells back into their pluripotent state to resemble embryonic stem cells. These cells are known as induced pluripotent stem cells. These cells are often obtained from taking a small sample from adult tissue, such as the skin. Because this process does not harm the donor nor does it involve embryos, the same ethical issues facing above-discussed cells are not encountered with induced pluripotent stem cells. Induced pluripotent stem cells can also reduce the risk of rejection by the body because the adult cells are derived from the person’s own body. Despite this exciting discovery, there are lots of obstacles to overcome before they are used in human studies.
Stem cell therapy is the use of stem cells to help replace or repair damaged tissue in the human body. In this type of therapy, stem cells are either differentiated into the cell of interest outside of the body prior to transplantation (exogenous), or directly transplanted into the site of injury (endogenous). In exogenous repair, scientists differentiate specific stem cell types in a laboratory and then implant the cells into the body. On the other hand, endogenous repair consists of directly transplanting stem cells into the body, and depends on the body’s ability to transform the cells into the specific type required for repair. These cells can be sourced from various places: autologous transplantation involves the use of stem cells from the person who is receiving the transplant, whereas allogenic transplantation involves receiving stem cells from a donor.
Cell transplantation versus stem cell transplantationCell transplantation is an umbrella term for stem cell transplantation. Different cells can be transplanted into the body including fat cells, bone marrow cells, and nose cells. Cell transplantation is the process of transferring cells into areas of the body that are damaged or injured. These transfers work in 2 main ways: 1) the cells can be transplanted into the injury site for direct repair, or 2) the cells can be transplanted into the blood where they can circulate around the body and cause system-wide changes. Most work in SCI focuses on putting a more specialized cell into the injury site (not just stem cells). For example, a stem cell can be pushed towards becoming a myelinating cell and then the myelinating cell could be transplanted into the injury site, or a Schwann cell could be harvested from the leg and then transplanted at the injury site. |
Currently, only a small number of conditions are approved to be treated with stem cell therapy. Bone marrow transplants for leukemia have been performed for many years. Epithelial stem cell transplants are also done to regenerate cells in those who have suffered burns or disorders of the cornea. Stem cell therapy for other purposes, including SCI, is still relatively new and much of the research is still in its early stages. The figure below highlights important landmarks in the history of stem cells research.
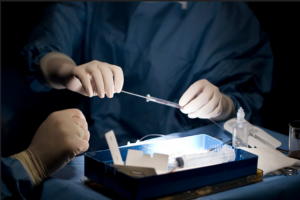
Healthcare professionals preparing for a stem cell transplant.18
With SCI, stem cells could theoretically be used to replace injured or destroyed nerve cells or protect further attack by the immune system around the injury site. The main goal of stem cell therapy for SCI is to improve the conduction of information past the injury. As of yet, there are no stem cell treatments that can reverse or repair a SCI. While there is some thought that stem cells may be used to help recovery, researchers are still currently unsure of exactly how stem cells/cell transplantation can help recovery from a spinal cord injury. However, many hypotheses have been made:
- Neuroprotection: Stem cells may help prevent further damage to the tissue in the area surrounding a spinal cord injury. By limiting the amount of damage, more function may be saved.
- Supporting the host cell: Stem cells may help enhance the survival of nearby cells by secreting molecules that control inflammation and/or improve the regeneration of blood cells
- Creating new blood vessels: Stem cells may help promote faster regrowth of new blood vessels and protection of the existing ones. Having more blood vessels may increase the amount of oxygen and nutrients available for surviving cells.
- Regrowth of axons: Stem cells may help axons regrow through developing bridges across the site of the lesion and decreasing scars. However, it is important to note that the regeneration of axons may not always lead to functional benefits and could even lead to adverse effects such as increased pain.
The anatomy of a neuron.19
- Regeneration of myelin: Myelin forms insulation around nerves, allowing electrical signals to transmit efficiently to other nerve cells. Stem cells may also be used to replace the support cells that wrap myelin around nerves.
Refer to our article on Neuroprotection for more information
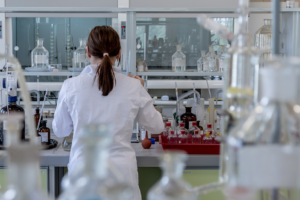
SCI research on stem cells has largely focused on laboratory and animal experiments. Human studies are in their initial phase.20
There are a number of ongoing and completed studies of weak to moderate evidence that evaluates the safety and efficacy of stem cells in people with SCI. Given that stem cells are still experimental, there is no specific stem cell type that is proven to be more effective over another. In research, studies have tested a large variation of stem cell sources, including blood, bone marrow, the nose, umbilical cords, and other nerves. While some of these stem cell sources come from fetuses, most studies have used stem cells from the person’s own body.
Care should be taken when interpreting these findings as they are derived from small trials with flaws in study design and documentation. Many more large-scale studies are required to determine whether stem cells have a role in treating people with SCI and if so, how transplantation should be done to minimize the health risks and maximize the potential of stem cell-based therapy.
Improved AIS score
In research, a common way to determine the success of any treatment is through comparing ASIA Impairment Scale (AIS) scores before and after transplantation. An individual’s AIS score consists of their muscle strength and sensation below their level of injury, in addition to anal sensation and contraction. Two weak evidence studies with a small number of participants (less than 10) found that stem cell treatments improved the AIS score of all participants. Conversely, two weak evidence studies found that only a portion (29-66.7%) of their participants saw an improvement in AIS scores.
Refer to our article on Spinal Cord Injury Basics for more information on AIS scores.
Natural recovery after SCIAlthough some studies have shown improvements in movement and sensory AIS scores, it is also important to note that natural (or spontaneous) recovery can occur within the first year of a spinal cord injury. In individuals with SCI, natural recovery of movement and sensation may occur up to 12-18 months post injury. Some stem cell studies have been conducted with newly injured individuals (i.e., less than 1 year after sustaining a spinal cord injury). As a result, spontaneous recovery may have played a role in the findings of improved function and sensation, not stem cell therapy. |
Improved sensation
There have been some mixed findings on the efficacy of stem cells on sensation. Some weak evidence research studies have seen improvements in sensation for some individuals, but no large-scale study has found improvements for their entire sample. A moderate evidence study found significant changes in sensation when compared to individuals who did not receive stem cell therapy. However, a second moderate evidence study found that sensation improved over a year, but the amount of improvement was not significantly different than individuals who did not receive stem cell therapy. Currently, it is hard to conclude whether the use of stem cells is effective for the restoration of sensation.
Improved motor function
There is some weak to moderate evidence showing that stem cells may help the recovery of motor function. The type of recovery in these studies has been quite diverse, ranging from a tetraplegic individual regaining the ability to hold their head up and move their upper limb, to the ability to walk with crutches and braces. However, the percentage of people who re-develop the ability to walk again in studies is low (i.e., 1-2 people out of 10-20 total participants). Moderate evidence suggests that stem cells may be more beneficial for movement in the arms and hands than the legs compared to people who did not receive stem cell therapy. Similar to sensation, no large-scale study has shown a high success rate in the recovery of motor functioning in response to stem cell therapy. In fact, there have been several studies that have found no improvements in motor function amongst their participants.
Improved neural connectivity
The signals relayed from the brain to the muscles are disrupted when a spinal cord injury occurs. To observe what connection remains, researchers apply stimulation to the brain and observe whether or not a signal is received at the arms/legs. If there is a signal present, researchers then note of how long it takes for the signal to get to the arms/legs, and the strength of the signal. As stem cells are supposed to theoretically help repair the spinal cord, it is thought that these signals would start to reappear/improve with stem cell therapy.
Motor evoked potentials
Motor evoked potentials are used to evaluate the connections between the brain and the muscles. Four low to moderate evidence studies have seen a reappearance of motor evoked potentials in individuals who were lacking them before, although the proportion of individuals who see a return is quite low (i.e., a range of 3-10 participants out of 20). Weak-moderate evidence research has also found improvements in the time it takes for signals to go through after stem cell therapy. It is important to note that these improvements are not always associated with improvements in AIS grades. Contrary to the previous findings, one research group found that the time taken for signals to travel from the brain to the muscle did not change, despite increases in AIS grade. This means that while functional improvements were seen, the connections between the brain and the muscle did not improve.
Somatosensory evoked potentials
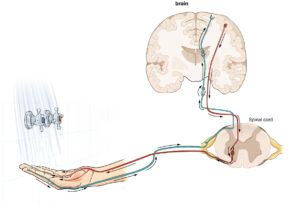
The brain normally interprets sensation through a pathway (in green) of nerves that travel from the limb, to the spinal cord, to the brain.24
are used to evaluate the sensory connections between the brain and the rest of the body. Several studies have found a reappearance of sensory signals in the wrist and/or ankles in a portion of their participants. For participants with sensory signals present prior to receiving stem cells, evidence shows that these signals may be strengthened, and that these signals may travel faster. Although these findings appear promising, the longevity of the strengthening effect is brought to light by one group of researchers who showed that the newly obtained sensory signals disappeared after one year. More research is required to determine how to consistently obtain these results, and to determine whether these effects are truly non-lasting.
Bladder & Bowel
Individuals with spinal cord injury often experience a lack of sensation and control of their bowel and bladder. Some research suggests that stem cell therapy may restore some sensation of bladder fullness and anal sensation. There are also some positive reports on the restoration of voluntary bladder contractions and anal control. Moreover, one study (moderate evidence) indicates that stem cell therapy may further improve bladder function by allowing individuals to hold more urine in their bladder, to increase the rate of urine passage, and to empty urine with less effort from by the bladder. However, the proportion of people in these studies who receive these bladder benefits are low.
Functional Independence and Quality of Life
There is limited evidence that stem cell therapy has a positive impact on functional independence and quality of life. Participants from three studies (weak evidence) reported that their self-care ability has improved in areas including bowel/bladder management, grooming, feeding, transferring, dressing, and mobility.
Sexual functioning
Most of the research on the effects of stem cell therapy and sexual functioning has been conducted with men. One weak study found that 31% of the men had improved erections while another found that sexual functioning improved as a result of increased sensitivity in the genital area. More research is required to determine the consistency of the effects in addition to the impact on sexual functioning of women.
Evidence for no improvement
While there have been some successful findings from stem cell therapy use in SCI, there are trials that have found no effects. Three studies found that none of their participants experienced any changes in their sensory or motor function. Meanwhile, other studies with a small number of participants (e.g., 5-20) have found that stem cell therapy may have an effect for some individuals, but not others. The number of people who do not experience any positive benefits from stem cell therapy is unpredictable, and has ranged from 40-87%.
Even though Health Canada and USA Food and Drug Administration (FDA) have not yet approved of any stem cell therapies for SCI in Canada, some private clinics around the world are already selling these treatments before establishing safety and effectiveness. In Canada, offering stem cell therapies is illegal unless it is for research purposes. Before you decide, consider the following points below with a health care provider. Use these points to think critically about what is being advertised.
There is no consensus on whether stem cell therapy is effective.
As described in this article, there is a lack of evidence supporting the use of stem cell therapy for spinal cord injury. Stem cell therapy is being explored as a treatment for many types of medical conditions; however, there is only strong evidence that supports its use with blood or immune system disorders that are often experienced after cancer. Currently, scientists are unsure how to repeatedly produce positive effects with the use of stem cell therapy for individuals with SCI. In addition, there remain many uncertain aspects of stem cell therapy such as knowing which type of stem cell is most effective for replacing different tissues, how these stem cells should be manipulated to produce the required cells, and what is the best way to deliver stem cells to the target area.
There are a variety of risks associated with stem cell therapy
Safety
There are many safety risks associated with stem cell treatments, and these risks may be higher for individuals with spinal cord injuries. Some stem cell marketers may emphasize that a treatment is safer if the cells are coming from your own body; however, this does not guarantee safety. If the stem cells are grown and multiplied before being injected into your spinal cord, the cells may lose some important properties and traits in the process, such as the ability to control growth. This may lead to the development of various tumors and/or cancers. In addition, when the cells are removed from your body, it is possible that they be exposed to bacteria or viruses prior to being re-transplanted into your spine. There is also a chance that complications may arise which may lead to short- or long-term health problems. This may lead to your injury or symptoms becoming more difficult to manage.
In clinical trials with people with SCI, there have been multiple adverse events resulting from stem cell therapy. The majority of stem cell studies conclude that transplantation is relatively safe due to the lack of serious safety problems or complications observed. Mild adverse events are infrequently reported in studies, but some have been documented. Some examples that have been reported include urinary tract infections, pain, hypothermia, headaches, increased spasticity, pressure sores, and depression. Serious adverse events that have been noted in research studies include:
-
- the worsening of motor functioning
- development of a cyst on the spine (which causes a concern for cancer)
- collection of fluid along the surgical site
- meningitis, resulting in a decrease in AIS score
- worsening of sensation (e.g., worsened symptoms of tingling, decrease in sensation)
However, it is important to note that these adverse effects were only monitored over the period of the study. Currently, long term effects and side effects of stem cell therapy is unknown. Further longitudinal research is required.
Financial
As the use of stem cell therapy is not currently approved by the government, most often government health programs and insurance companies will not cover the costs. The costs of receiving stem cell therapy can be very high. On top of the cost for the treatment (being in the tens of thousands of dollars itself), there is the cost of travel, accommodations, and other fees. In addition, travelling for stem cell therapy may require you to go through a medical procedure without the support of all your family and friends.
Research
Receiving an experimental treatment is not the same as participating in a clinical trial. If you choose to receive stem cell therapy from a private clinic, you may be ineligible to participate in future clinical trials. Participating in a clinical trial is advised over receiving stem cell therapy from an unregulated private clinic, as:
-
- Clinical trials are overseen by an independent medical ethics committee. This group of people is there to protect your rights and safety as a participant.
- Clinical trials require some safety and efficacy testing before trying it in humans. This ensures that the treatment is relatively safe and effective.
- By participating in a clinical trial (research), you are contributing back to the pool of knowledge. When you partake in a research study, you help scientists learn more about stem cell therapy and the safety or effectiveness of it.
- There is no cost for the treatment in a clinical trial, as participants are not required to pay to partake in research studies.
Stem cell marketing sites are likely misleading
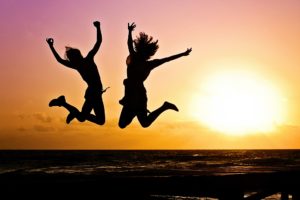
Private stem cell clinics often use overly positive/ optimistic advertisements that may be misleading.31
Unapproved stem cell marking websites often feature many patient testimonials in addition to references to research studies. The use of testimonials may be very persuasive, but may be misleading. Some of the positive benefits that these individuals may be feeling may be the result of the placebo effect. Given that many people who go in for stem cell therapy treatment have a strong belief that it will work, they may perceive less pain or greater sensation after their treatment. Patient anecdotes should not be held equal to research evidence supporting the use of stem cell therapy.
Additionally, many private stem cell therapy clinics will try to sell the treatment by citing multiple research studies to build credibility. In reality, these research articles often have no direct association with stem cell therapy specific to spinal cord injuries. Currently, there is a lack of high-quality research studies on stem cell therapy. As a result, many of the human-based studies cited are low quality and are not specific to spinal cord injury, and some reports are based on animal trials.
Before taking part in a research trial, it is important to seek medical advice from your healthcare team. Your healthcare providers can direct you to SCI studies that are available, assess whether you are a suitable candidate, and provide you with educational resources. There are also online databases containing registered clinical trials such as SciTrialsFinder.net, ClinicalTrials.gov, ISRCTN Registry, and the International Clinical Trials Registry Platform (ICTRP).
Ensure that the trial you wish to partake in is approved by:
- An independent review committee such as an Institutional Review Board (IRB) or Ethics Review Board (ERB) to protect your rights as a participant
- A regulatory agency such as Health Canada, USA Food and Drug Administration (FDA), or European Medicines Agency (EMEA) to make sure the treatment will be conducted in a safe manner
Experimental Treatments for Spinal Cord Injury: What you should know is a guide that outlines other important considerations to think about before agreeing to participate in a clinical trial for an experimental treatment.
Stem cells continue to be a hot topic in SCI research. Their therapeutic potential in preventing further damage to the spinal cord and promoting regrowth of nerve cells has inspired a vast number of studies. However, it is important to note that there is a lack of consistency in the research in regards to the effectiveness and reported benefits of stem cell therapy for individuals with SCI. While there has been some positive evidence for stem cell therapy in these studies, it remains unclear whether this treatment is safe and effective for people with SCI.
There are currently no approved stem cell treatments for SCI. Stem cell treatments have not been proven to be safe and effective in clinical trials. Even so, many clinics around the world offer stem cell therapies that do not have proper evidence to back up their claims. Although stem cells therapy is taking a long time to get approved as a regulated treatment, remember that the process by which research is translated into medical practice is in place to minimize harms and to maximize benefits and effectiveness. If you wish to undergo a stem cell treatment, the risks should be weighed against the benefits. It is best to discuss all treatment options with your health providers to find out which treatments are suitable for you.
For a review of how we assess evidence at SCIRE Community and advice on making decisions, please see SCIRE Community Evidence.
Evidence for “What are stem cells” is based on:
Canadian Stem Cell Foundation (2016). About Spinal Cord Injury. 1–4.
Evidence for “What are stem cells used for” is based on:
Insoo, H. (2010). The bioethics of stem cell research and therapy. Journal of Clinical Investigation. https://doi.org/10.1172/jci40435
International Society for Stem Cell Research. (2008). Patient Handbook on Stem Cell Therapies. 8.
Science learning hub. (2016). Stem cell research – timeline. Retreived from: https://sciencelearn.org.nz/resources/1967-stem-cell-research-timeline
What is biotechnology? (n.d.). Stem cells. Retrieved from: https://whatisbiotechnology.org/index.php/science/summary/stem/stem-cells-repair-tissues-and-regenerate-cells
New Scientist. (2014). Stem cell timeline: the history of a medical sensation. Retreived from: https://newscientist.com/article/dn24970-stem-cell-timeline-the-history-of-a-medical-sensation/
Evidence for “How can stem cells be used for SCI” is based on:
Assinck, P., Duncan, G. J., Hilton, B. J., Plemel, J. R., & Tetzlaff, W. (2017). Cell transplantation therapy for spinal cord injury. Nature Neuroscience, 20(5), 637–647. https://doi.org/10.1038/nn.4541
Evidence for “What stem cell research has been done in SCI” is based on:
Al-Zoubi, A., Jafar, E., Jamous, M., Al-Twal, F., Al-Bakheet, S., Zalloum, M., … Al-Zoubi, Z. (2014). Transplantation of Purified Autologous Leukapheresis-Derived CD34 + and CD133 + Stem Cells for Patients with Chronic Spinal Cord Injuries: Long-Term Evaluation of Safety and Efficacy . Cell Transplantation, 23(1_suppl), 25–34. https://doi.org/10.3727/096368914×684899
Cheng, H., Liu, X., Hua, R., Dai, G., Wang, X., Gao, J., & An, Y. (2014). Clinical observation of umbilical cord mesenchymal stem cell transplantation in treatment for sequelae of thoracolumbar spinal cord injury.https://doi.org/10.1186/s12967-014-0253-7
Chotivichit, A., Ruangchainikom, M., Chiewvit, P., Wongkajornsilp, A., & Sujirattanawimol, K. (2015). Chronic spinal cord injury treated with transplanted autologous bone marrow-derived mesenchymal stem cells tracked by magnetic resonance imaging: a case report. https://doi.org/10.1186/s13256-015-0535-6
Cristante, A. F., Barros-Filho, T., Tatsui, N., Mendrone, A., Caldas, J. G., Camargo, A., … Marcon, R. M. (2009). Stem cells in the treatment of chronic spinal cord injury: evaluation of somatosensitive evoked potentials in 39 patients. Spinal Cord, 47, 733–738. https://doi.org/10.1038/sc.2009.24
Dai, G., Liu, X., Zhang, Z., Wang, X., Li, M., Cheng, H., … An, Y. (2013). Comparative analysis of curative effect of CT-guided stem cell transplantation and open surgical transplantation for sequelae of spinal cord injury. Retrieved from http://www.translational-medicine.com/content/11/1/315
Deda, H., Kürekçi, A. E., Kayıhan, K., Zgün, E. O. ¨, Stünsoy, U. ¨, & Kocabay, S. (2008). Treatment of chronic spinal cord injured patients with autologous bone marrow-derived hematopoietic stem cell transplantation: 1-year follow-up. Cytotherapy, 10(6), 565–574. https://doi.org/10.1080/14653240802241797
Fawcett, J.W., Curt, A., Steeves, J.D., Coleman, W.P.,Tuszynski, M.H., Lammertse, D…& Short, D. (2007). Guidelines for the conduct of clinical trials for spinal cord injury as developed by the ICCP panel: spontaneous recovery after spinal cord injury and statistical power needed for therapeutic clinical trials. Spinal cord, 45(3), 190-205. doi:10.1038/sj.sc.3102007
Frolov, A. A., & Bryukhovetskiy, A. S. (2012). Effects of Hematopoietic Autologous Stem Cell Transplantation to the Chronically Injured Human Spinal Cord Evaluated by Motor and Somatosensory Evoked Potentials Methods. Cell Transplantation, 21, 49–55. https://doi.org/10.3727/096368912X633761
Jarocha, D., Milczarek, O., Kawecki, Z., Wendrychowicz, A., Kwiatkowski, S., & Majka, M. (2014). Preliminary study of autologous bone marrow nucleated cells transplantation in children with spinal cord injury. Stem Cells Translational Medicine, 3(3), 395–404. https://doi.org/10.5966/sctm.2013-0141
Jarocha, D., Milczarek, O., Wedrychowicz, A., Kwiatkowski, S., & Majka, M. (2015). Continuous improvement after multiple mesenchymal stem cell transplantations in a patient with complete spinal cord injury. Cell Transplantation, 24(4), 661–672. https://doi.org/10.3727/096368915X687796
Kang, K.-S., Kim, S. W., Oh, Y. H., Yu, J. W., Kim, K.-Y., Park, H. K., … Han, H. (2005). A 37-year-old spinal cord-injured female patient, transplanted of multipotent stem cells from human UC blood, with improved sensory perception and mobility, both functionally and morphologically: a case study. International Society for Cellular Therapy, 7(4), 368–373. https://doi.org/10.1080/14653240500238160
Liu, J., Han, D., Wang, Z., Xue, M., Zhu, L., Yan, H., … Wang, & H. (2013). Clinical analysis of the treatment of spinal cord injury with umbilical cord mesenchymal stem cells. Cytotherapy, 15, 185–191. https://doi.org/10.1016/j.jcyt.2012.09.005
Moviglia, G. A., Fernandez Viña, R., Brizuela, J. A., Saslavsky, J., Vrsalovic, F., Varela, G., … Shuster, G. S. (2006). Combined protocol of cell therapy for chronic spinal cord injury. Report on the electrical and functional recovery of two patients. Cytotherapy. https://doi.org/10.1080/14653240600736048
Oh, S. K., Choi, K. H., Yoo, J. Y., Kim, D. Y., Kim, S. J., & Jeon, S. R. (2016). A Phase III Clinical Trial Showing Limited Efficacy of Autologous Mesenchymal Stem Cell Therapy for Spinal Cord Injury. Neurosurgery, 78(3), 436–447. https://doi.org/10.1227/NEU.0000000000001056
Oraee-Yazdani, S., Hafizi, M., Atashi, A., Ashrafi, F., Seddighi, A.-S., Hashemi, S. M., … Zali, A. (2016). Co-transplantation of autologous bone marrow mesenchymal stem cells and Schwann cells through cerebral spinal fluid for the treatment of patients with chronic spinal cord injury: safety and possible outcome. https://doi.org/10.1038/sc.2015.142
Park, H. C., Shim, Y. S., Ha, Y., Yoon, S. H., Park, S. R., Choi, B. H., & Park, H. S. (2005). Treatment of complete spinal cord injury patients by autologous bone marrow cell transplantation and administration of granulocyte-macrophage colony stimulating factor. Tissue Engineering, 11(5–6), 913–922. https://doi.org/10.1089/ten.2005.11.913
Park, J. H., Kim, D. Y., Sung, I. Y., Choi, G. H., Jeon, M. H., Kim, K. K., & Jeon, S. R. (2012). Long-term results of spinal cord injury therapy using mesenchymal stem cells derived from bone marrow in humans. Neurosurgery, 70(5), 1238–1247. https://doi.org/10.1227/NEU.0b013e31824387f9
Shin, J. C., Kim, K. N., Yoo, J., Kim, I. S., Yun, S., Lee, H., … Park, K. I. (2015). Clinical Trial of Human Fetal Brain-Derived Neural Stem/Progenitor Cell Transplantation in Patients with Traumatic Cervical Spinal Cord Injury. Neural Plasticity, 2015. https://doi.org/10.1155/2015/630932
Vaquero, J., Zurita, M., Rico, M. A., Bonilla, C., Aguayo, C., Montilla, J., … Reina, L. De. (2016). An approach to personalized cell therapy in chronic complete paraplegia: The Puerta de Hierro phase I/II clinical trial. Cytotherapy, 18(8), 1025–1036. https://doi.org/10.1016/j.jcyt.2016.05.003
Vaquero, J., Zurita, M., Rico, M. A., Bonilla, C., Aguayo, C., Fernández, C., … Fernández, M. V. (2017). Repeated subarachnoid administrations of autologous mesenchymal stromal cells supported in autologous plasma improve quality of life in patients suffering incomplete spinal cord injury. Cytotherapy, 19, 349–359. https://doi.org/10.1016/j.jcyt.2016.12.002
Yazdani, S. O., Hafizi, M., Zali, A. R., Atashi, A., Ashrafi, F., Seddighi, A. S., & Soleimani, M. (2013). Safety and possible outcome assessment of autologous Schwann cell and bone marrow mesenchymal stromal cell co-transplantation for treatment of patients with chronic spinal cord injury. Cytotherapy. https://doi.org/10.1016/j.jcyt.2013.03.012
Evidence for “What are the risks and considerations of using stem cells” is based on:
A Closer Look at Stem Cells. (2019). Nine things to know about stem cell treatments. Retreived from: https://closerlookatstemcells.org/stem-cells-medicine/nine-things-to-know-about-stem-cell-treatments/
Al-Zoubi, A., Jafar, E., Jamous, M., Al-Twal, F., Al-Bakheet, S., Zalloum, M., … Al-Zoubi, Z. (2014). Transplantation of Purified Autologous Leukapheresis-Derived CD34 + and CD133 + Stem Cells for Patients with Chronic Spinal Cord Injuries: Long-Term Evaluation of Safety and Efficacy . Cell Transplantation, 23(1_suppl), 25–34. https://doi.org/10.3727/096368914×684899
Anderson, K. D., Guest, J. D., Dietrich, W. D., Bunge, M. B., Curiel, R., Dididze, M., … Levi, A. D. (2017). Safety of Autologous Human Schwann Cell Transplantation in Subacute Thoracic Spinal Cord Injury. Journal of Neurotrauma, 34, 2950–2963. https://doi.org/10.1089/neu.2016.4895
Assinck, P., Duncan, G. J., Hilton, B. J., Plemel, J. R., & Tetzlaff, W. (2017). Cell transplantation therapy for spinal cord injury. Nature Neuroscience, 20(5), 637–647. https://doi.org/10.1038/nn.4541
Blight, A., Curt, A., Ditunno, J. F., Dobkin, B., Ellaway, P., Fawcett, J., … Guest, J. D. (2009). Position statement on the sale of unproven cellular therapies for spinal cord injury: The international campaign for cures of spinal cord injury paralysis. Spinal Cord, 47(9), 713–714. https://doi.org/10.1038/sc.2008.179
Cheng, H., Liu, X., Hua, R., Dai, G., Wang, X., Gao, J., & An, Y. (2014). Clinical observation of umbilical cord mesenchymal stem cell transplantation in treatment for sequelae of thoracolumbar spinal cord injury. https://doi.org/10.1186/s12967-014-0253-7
Chotivichit, A., Ruangchainikom, M., Chiewvit, P., Wongkajornsilp, A., & Sujirattanawimol, K. (2015). Chronic spinal cord injury treated with transplanted autologous bone marrow-derived mesenchymal stem cells tracked by magnetic resonance imaging: a case report. ??? https://doi.org/10.1186/s13256-015-0535-6
Cristante, A. F., Barros-Filho, T., Tatsui, N., Mendrone, A., Caldas, J. G., Camargo, A., … Marcon, R. M. (2009). Stem cells in the treatment of chronic spinal cord injury: evaluation of somatosensitive evoked potentials in 39 patients. Spinal Cord, 47, 733–738. https://doi.org/10.1038/sc.2009.24
Curt, A., Hsieh, J., Schubert, M., Hupp, M., Friedl, S., Freund, P., … Guzman, R. (2019). Safety and preliminary efficacy of allogenic neural stem cell transplantation in chronic spinal cord injury: A translational phase I/IIa trial. Preprints with the Lancet, 73, 1–21.
Dai, G., Liu, X., Zhang, Z., Wang, X., Li, M., Cheng, H., … An, Y. (2013). Comparative analysis of curative effect of CT-guided stem cell transplantation and open surgical transplantation for sequelae of spinal cord injury. Retrieved from http://www.translational-medicine.com/content/11/1/315
Deda, H., Kürekçi, A. E., Kayıhan, K., Zgün, E. O. ¨, Stünsoy, U. ¨, & Kocabay, S. (2008). Treatment of chronic spinal cord injured patients with autologous bone marrow-derived hematopoietic stem cell transplantation: 1-year follow-up. Cytotherapy, 10(6), 565–574. https://doi.org/10.1080/14653240802241797
Dlouhy, B. J., Awe, O., Rao, R. C., Kirby, P. A., & Hitchon, P. W. (2014). Autograft-derived spinal cord mass following olfactory mucosal cell transplantation in a spinal cord injury patient. Journal of Neurosurgery: Spine, 21(4), 618–622. https://doi.org/10.3171/2014.5.SPINE13992
Fawcett, J. W., Curt, A., Steeves, J. D., Coleman, W. P., Tuszynski, M. H., Lammertse, D., … Short, D. (2007). Guidelines for the conduct of clinical trials for spinal cord injury as developed by the ICCP panel: Spontaneous recovery after spinal cord injury and statistical power needed for therapeutic clinical trials. Spinal Cord, 45(3), 190–205. https://doi.org/10.1038/sj.sc.3102007
Féron, F., Perry, C., Cochrane, J., Licina, P., Nowitzke, A., Urquhart, S., … Mackay-Sim, A. (2005). Autologous olfactory ensheathing cell transplantation in human spinal cord injury. Brain : A Journal of Neurology, 128(Pt 12), 2951–2960. https://doi.org/10.1093/brain/awh657
Foundation, C. S. C. (2016). About Spinal Cord Injury. 1–4.
Frolov, A. A., & Bryukhovetskiy, A. S. (2012). Effects of Hematopoietic Autologous Stem Cell Transplantation to the Chronically Injured Human Spinal Cord Evaluated by Motor and Somatosensory Evoked Potentials Methods. Cell Transplantation, 21, 49–55. https://doi.org/10.3727/096368912X633761
Insoo, H. (2010). The bioethics of stem cell research and therapy. Journal of Clinical Investigation. https://doi.org/10.1172/jci40435
International Society for Stem Cell Research. (2008). Patient Handbook on Stem Cell Therapies. 8.
International Society for Stem Cell Research. (2020). Nine Things To Know About Stem Cell Treatments – A Closer Look at Stem Cells. Retrieved April 9, 2020, from https://www.closerlookatstemcells.org/stem-cells-medicine/nine-things-to-know-about-stem-cell-treatments/
Jarocha, D., Milczarek, O., Kawecki, Z., Wendrychowicz, A., Kwiatkowski, S., & Majka, M. (2014). Preliminary study of autologous bone marrow nucleated cells transplantation in children with spinal cord injury. Stem Cells Translational Medicine, 3(3), 395–404. https://doi.org/10.5966/sctm.2013-0141
Jarocha, D., Milczarek, O., Wedrychowicz, A., Kwiatkowski, S., & Majka, M. (2015). Continuous improvement after multiple mesenchymal stem cell transplantations in a patient with complete spinal cord injury. Cell Transplantation, 24(4), 661–672. https://doi.org/10.3727/096368915X687796
Kang, K.-S., Kim, S. W., Oh, Y. H., Yu, J. W., Kim, K.-Y., Park, H. K., … Han, H. (2005). A 37-year-old spinal cord-injured female patient, transplanted of multipotent stem cells from human UC blood, with improved sensory perception and mobility, both functionally and morphologically: a case study. International Society for Cellular Therapy, 7(4), 368–373. https://doi.org/10.1080/14653240500238160
Lima, C., Escada, P., Pratas-Vital, J., Branco, C., Arcangeli, C. A., Lazzeri, G., … Peduzzi, J. D. (2010). Olfactory Mucosal Autografts and Rehabilitation for Chronic Traumatic Spinal Cord Injury. Neurorehabilitation and Neural Repair, 24(1), 10–22. https://doi.org/10.1177/1545968309347685
Lima, C., Pratas-Vital, J., Escada, P., Hasse-Ferreira, A., Capucho, C., & Peduzzi, J. D. (2006). Olfactory mucosa autografts in human spinal cord injury: A pilot clinical study. Journal of Spinal Cord Medicine, 29(3), 191–203. https://doi.org/10.1080/10790268.2006.11753874
Liu, J., Han, D., Wang, Z., Xue, M., Zhu, L., Yan, H., … Wang, & H. (2013). Clinical analysis of the treatment of spinal cord injury with umbilical cord mesenchymal stem cells. Cytotherapy, 15, 185–191. https://doi.org/10.1016/j.jcyt.2012.09.005
Mackay-Sim, A., Feron, F., Cochrane, J., Bassingthwaighte, L., Bayliss, C., Davies, W., … Geraghty, T. (2008). Autologous olfactory ensheathing cell transplantation in human paraplegia: a 3-year clinical trial. Brain, 131, 2376–2386.
Master, Z., & Caulfield, T. (2014). What you need to know about stem cell therapies. Retrieved from https://oirm.ca/sites/default/files/about-orim/sc_patient_booklet_feb_2014.pdf
Moviglia, G. A., Fernandez Viña, R., Brizuela, J. A., Saslavsky, J., Vrsalovic, F., Varela, G., … Shuster, G. S. (2006). Combined protocol of cell therapy for chronic spinal cord injury. Report on the electrical and functional recovery of two patients. Cytotherapy. https://doi.org/10.1080/14653240600736048
Oh, S. K., Choi, K. H., Yoo, J. Y., Kim, D. Y., Kim, S. J., & Jeon, S. R. (2016). A Phase III Clinical Trial Showing Limited Efficacy of Autologous Mesenchymal Stem Cell Therapy for Spinal Cord Injury. Neurosurgery, 78(3), 436–447. https://doi.org/10.1227/NEU.0000000000001056
Oraee-Yazdani, S., Hafizi, M., Atashi, A., Ashrafi, F., Seddighi, A.-S., Hashemi, S. M., … Zali, A. (2016). Co-transplantation of autologous bone marrow mesenchymal stem cells and Schwann cells through cerebral spinal fluid for the treatment of patients with chronic spinal cord injury: safety and possible outcome. https://doi.org/10.1038/sc.2015.142
Park, H. C., Shim, Y. S., Ha, Y., Yoon, S. H., Park, S. R., Choi, B. H., & Park, H. S. (2005). Treatment of complete spinal cord injury patients by autologous bone marrow cell transplantation and administration of granulocyte-macrophage colony stimulating factor. Tissue Engineering, 11(5–6), 913–922. https://doi.org/10.1089/ten.2005.11.913
Park, J. H., Kim, D. Y., Sung, I. Y., Choi, G. H., Jeon, M. H., Kim, K. K., & Jeon, S. R. (2012). Long-term results of spinal cord injury therapy using mesenchymal stem cells derived from bone marrow in humans. Neurosurgery, 70(5), 1238–1247. https://doi.org/10.1227/NEU.0b013e31824387f9
Rao, Y., Zhu, W., Liu, H., Jia, C., Zhao, Q., & Wang, Y. (2013). Clinical application of olfactory ensheathing cells in the treatment of spinal cord injury. Journal of International Medical Research. https://doi.org/10.1177/0300060513476426
Shin, J. C., Kim, K. N., Yoo, J., Kim, I. S., Yun, S., Lee, H., … Park, K. I. (2015). Clinical Trial of Human Fetal Brain-Derived Neural Stem/Progenitor Cell Transplantation in Patients with Traumatic Cervical Spinal Cord Injury. Neural Plasticity, 2015. https://doi.org/10.1155/2015/630932
Sipski, M. L., & Arenas, A. (2006). Female sexual function after spinal cord injury. Progress in Brain Research. https://doi.org/10.1016/S0079-6123(05)52030-2
Vaquero, J., Zurita, M., Rico, M. A., Bonilla, C., Aguayo, C., Fernández, C., … Fernández, M. V. (2017). Repeated subarachnoid administrations of autologous mesenchymal stromal cells supported in autologous plasma improve quality of life in patients suffering incomplete spinal cord injury. Cytotherapy, 19, 349–359. https://doi.org/10.1016/j.jcyt.2016.12.002
Vaquero, J., Zurita, M., Rico, M. A., Bonilla, C., Aguayo, C., Montilla, J., … Reina, L. De. (2016). An approach to personalized cell therapy in chronic complete paraplegia: The Puerta de Hierro phase I/II clinical trial. Cytotherapy, 18(8), 1025–1036. https://doi.org/10.1016/j.jcyt.2016.05.003
Yazdani, S. O., Hafizi, M., Zali, A. R., Atashi, A., Ashrafi, F., Seddighi, A. S., & Soleimani, M. (2013). Safety and possible outcome assessment of autologous Schwann cell and bone marrow mesenchymal stromal cell co-transplantation for treatment of patients with chronic spinal cord injury. Cytotherapy. https://doi.org/10.1016/j.jcyt.2013.03.012
Image credits
- Embryonic Stem Cell ©Gorkem Oner, CC BY 3.0 US
- Human Embryonic Stem Cells ©Russo E, CC BY 2.5
- Stem cell ©DailyPM, CC BY 3.0 US
- Rat ©Ayub Irawan, CC BY 3.0 US
- Waving ©Adrien Coquet, CC BY 3.0 US
- Spine ©Sahua D, CC BY 3.0 US
- Heart ©Alfa deisgn, CC BY 3.0 US
- Blind ©Adrien Coquet, CC BY 3.0 US
- Idea ©ibrandify, CC BY 3.0 US
- Bone ©Saeful Muslim, CC BY 3.0 US
- Liver ©Fauzan, CC BY 3.0 US
- Blood ©Evgeny Filatov, CC BY 3.0 US
- Eye ©Harpal Singh, CC BY 3.0 US
- White Cells ©Prosymbols, CC BY 3.0 US
- Brain ©Redouane Sayah, CC BY 3.0 US
- Person ©Andre Ru, CC BY 3.0 US
- Human ©Andrejs Kirma, CC BY 3.0 US
- Stem cells ©Bryan Jones, CC BY-NC-ND 2.0
- Neuron ©US National Cancer Institute’s Surveillance, Epidemiology, and End Results Program, CC BY-SA 3.0
- Research ©jarmoluk, CC0 1.0
- Neuropathy ©Servier Medical Art, CC BY 3.0
- Silhouette walking crutches man ©Mohamed Hassan, Pixabay
- Neuron brain nervous system neurons nerve cell CC0 1.0
- Sensory nervous system activation and response ©Openstax CC BY 4.0
- Bladder ©fauzan akbar, CC BY 3.0 US
- Large intestine ©BomSymbols, CC BY 3.0 US
- Transferring out of car. © The SCIRE Team
- Warning ©Oliver Silvérus, CC BY 3.0 US
- Money ©binpodo, CC BY 3.0 US
- Research ©Tezar Tantular, CC BY 3.0 US
- Youth active jump happy sunrise silhouettes two ©JillWellington, Pixabay