Author: Sharon Jang | Reviewer: Riley Louie | Published: 7 April 2021 | Updated: ~
New technology has led to the creation of wearable robotic devices to improve leg movement for activities such as standing and walking. This page discusses the use of robotic exoskeletons in people after spinal cord injury (SCI).
Key Points
- Robotic exoskeletons are electromechanical devices that are worn around the limbs to support activities such as standing and walking in SCI people.
- In addition to improved mobility, robotic exoskeletons allow increased levels of activity for general health benefits related to regular exercise.
- Limitations of using exoskeletons include their high costs, limited availability, and restricted use in real-world settings.
- Moderate evidence shows that exoskeletons increase safety and decrease energy requirements during walking for people with thoracic SCI.
Robotic exoskeletons (also known as powered gait orthoses) are wearable electromechanical devices that enhance movement of weak or paralyzed legs. They are electrically powered at the joints, allowing the hips, knees, and ankles to move. The greatest advantage exoskeletons have over passive orthotics or braces is that they are programmed to enable coordinated movement without much effort from the user. This is especially so since exoskeletons carry their own weight as well as that of the user. Movements that most exoskeletons assist include sit-to-stand and walking.
As of 2019, many different models of exoskeletons exist, and this number continues to grow as research and technology progresses. However, it is important to note that only three have been approved by the FDA for sale in North America and only two have been approved for home use, while the other is only approved for research and rehabilitation purposes. The two that are approved for home use are the ReWalk and the Indego, while the Ekso has been approved for research and rehabilitation purposes. The current models of exoskeletons have the ability to move from 0.2-2.6 km/hr, and weigh between 12-38 kg.
Exoskeletons are slowly moving into the community. Currently, most models can only move over flat, smooth surfaces. However, some can go up inclines. Moreover, newer models have the added function of enabling the user to sit in or wheel a wheelchair without having to take off the device. These features can increase the independence of people with SCI and enable/enhance the performance of activities such as standing, walking, and climbing stairs. However, these devices are still expensive, ranging from $70,000-120,000 USD.
In deciding if robotic exoskeletons are an appropriate option for you, a health care provider will perform an assessment where they account for factors such as your level of injury, risk for falls and fractures, and range of movement. Once exoskeletons are deemed appropriate, training will be required to learn how to properly use the device. A physiotherapist or caregiver may help you put on and take off the device. Generally, the feet are placed on footplates and the torso, hips, and legs are strapped into the exoskeleton. Some models extend upwards to include a backpack-like structure, which offers more trunk support and contains the computer and battery. Other models are secured only at the waist and below. In addition, some models can be programmed externally using a tablet. A pair of forearm crutches or a walker is often used to maintain balance. As someone with SCI walks, the built-in sensors and motorized joints (hip, knee, and/or ankle) constantly accommodate to encourage a rhythmic walking pattern. When the individual improves their own walking function and requires less assistance, the exoskeleton can be adjusted to provide less support.
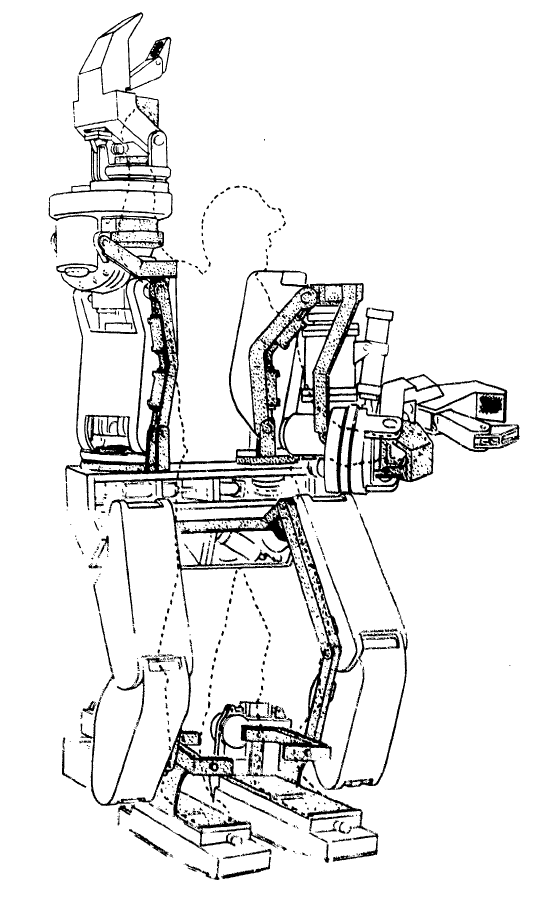
A conceptual diagram of the Hardiman.
The term “exoskeleton” was originally borrowed from animal biology. An exoskeleton (such as a shell) is an outer cover that protects and supports the animal. In a similar way, robotic exoskeletons have been designed to help externally support and enhance human movement.
The earliest exoskeletons were developed with a military focus, aimed to help soldiers carry heavier loads, run faster, and jump higher. In 1968, the first exoskeleton, dubbed “the Hardiman” was developed in partnership with the US military. This exoskeleton was originally designed to help amplify soldiers’ strength by 25 times (i.e., lifting a 1500 item would feel like you are lifting a 60 lb item). Although the Hardiman was created and worked, it was not without limitations. First of all, the exoskeleton itself weighed 1500 lb. It was hydraulically-powered and required pumps and bladders that could fill a room. Despite its abilities, it was not very functional.
In 1972, a team from Yugoslavia developed the first functional exoskeleton: the kinematic walker. This device was the first of its kind; a powered robot consisting of a single hydraulic actuator (motor), which reduced its size. The kinematic walker was designed as a walking orthotic, and allowed for smooth movements to be made. Some movements the exoskeleton was able to perform included flexion and extension of the hip, knee, and ankle, in addition to abduction and adduction of the legs. Although this exoskeleton only weighed 12 kg, it required a separate power source and a computer, which were externally located from the exoskeleton.
For more information on treadmill-based robotics, refer to our articles on Body Weight Supported Treadmill Training and Functional Electrical Stimulation for more information on treadmill based robotics.
In 2001, the first exoskeleton products started being sold. The Lokomat, a robotic exoskeleton that was suspended over a treadmill, was one of the first exoskeletons used for rehabilitation. Meanwhile, the US Defense Advanced Research Projects Agency (DARPA) had announced their Human Performance Augmentation program which offered funding to develop exoskeletons for military use. From this funding, two separate groups developed exoskeletons – the Berkeley Lower Extremity Exoskeleton (BLEEX) and the Raytheon XOS suit. Both suits were created to help soldiers carry extra weight (up to 200 lb!) without feeling it.
Starting in 2010, more rehabilitation-based exoskeletons started entering the market. These include gait-assistive devices commonly seen in the media today, such as the Ekso, the ReWalk, and the Indego exoskeletons. These models are described in the section below
There are many types of exoskeletons that are currently available for use for individuals with SCI. While some exoskeletons have only been cleared for rehabilitation purposes, others have separate models for personal or home use. Below we discuss the general differences between rehabilitation and personal exoskeletons.
Rehabilitation Exoskeletons
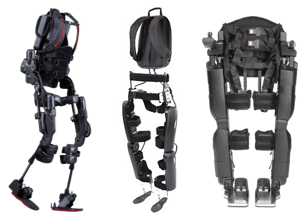
The Ekso (left), the ReWalk (middle), and the REX (right) exoskeletons.4-6
Exoskeletons used for rehabilitation are generally heavier than personal exoskeletons and are often controlled by a computer and battery located in a backpack worn by the user. However, rehabilitation exoskeletons often come with more customizable abilities. For example, the Ekso and the ReWalk allow the clinician to modify the amount of power (i.e., support from the robot) for each leg, depending on the ability of the user. Similarly, the therapy version of the Indego allows the clinician to control how much weight is supported by the exoskeleton and how much movement assistance is provided by the exoskeleton. Moreover, how steps are triggered can be programmed in different ways: e.g., by the press of a button, by shifting your body weight, or by initiating a step using your own muscles. These bulkier clinical models are usually one-size-fits-all, allowing clinicians to adjust the dimensions of the device (i.e., leg length, hip width, etc.) to treat their clients of varying body sizes.
The REX differs from all of the aforementioned exoskeletons in that it is controlled with a joystick and is able to self-balance, making it essentially “hands-free”. This feature allows individuals to perform exercises while standing, including squats, lunges, and upper body exercises using both hands.
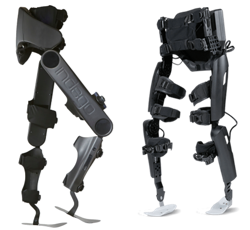
The Indego (left) and the ReWalk (right).
Personal Exoskeletons
Exoskeletons that have been designed for home use are generally lighter and provide limited support to the torso. Personal exoskeletons have a battery pack that is connected to the waist, but otherwise operates similarly to the rehabilitation models in that a weight shift initiates a step. Furthermore, some exoskeleton companies have developed apps that accompany the exoskeleton, allowing the user to independently access performance data. These personal exoskeletons are usually custom-developed to fit the user’s unique body dimensions.
While there are many purported benefits of using robotic exoskeletons among individuals with SCI, strong research evidence is lacking. Exoskeletons are largely used to enhance mobility in SCI, where they can be used for walk (gait) training during rehabilitation or in the community (at home) to perform simple daily activities. Although further research is required, some research has suggested that walking with an exoskeleton may have additional health benefits:
Spasticity
There are mixed findings regarding the effects of exoskeletal walking on spasticity. Many (weak evidence) studies have found that using exoskeletons can result in decreased spasticity. Despite these noted benefits, one weak evidence study reported mixed findings on spasticity as 26.7% of their study sample saw a decrease in spasticity, while 62.2% of participants saw no change and 11.1% saw an increase in spasticity. The impact of exoskeletal walking on spasticity may be related to the user’s baseline level of spasticity. In a weak evidence study, users who had low levels of spasticity prior to using an exoskeleton experienced an increase in spasticity; however, this increase in spasticity ultimately decreased over 12 weeks back to near zero. Individuals who already had high levels of spasticity prior to walking in an exoskeleton saw no changes in spasticity.
Bowel function
There is some weak research evidence that suggests walking with an exoskeleton can help with various bowel functions, including improved regularity of bowel movements, less time required for bowel management, and decreased enema dose. However, two (weak evidence) studies have found no effects on bowel function.
Bone Health
After SCI, bone mineral density in the legs declines at rapid rates due to inactivity and weight bearing activities have the potential to help restore bone mineral density. One study (weak evidence) found that walking in an exoskeleton may increase bone mineral density up to 14% with 6 weeks of training.
Fitness
Some research (weak evidence) suggests that walking in an exoskeleton can provide good exercise for the heart and upper and lower limb strength in those with incomplete SCI. More details about the effect of exoskeletons on fitness are discussed below in the section: “Can I get exercise benefits from walking in an exoskeleton?”
Pain
A few studies (weak evidence) report a decrease in pain with exoskeleton use, with one noting a reduction in pain, but not enough to significantly impact everyday life (i.e., clinical significance). On the other hand, some weak evidence studies have found no effect of exoskeleton walking on pain.
Pressure Sores
There is some weak research evidence that walking in an exoskeleton may help avoid the negative effects of prolonged standing or sitting (e.g., pressure ulcers).
Refer to our articles on Pressure Sores, Pain and Spasticity for more information!
While a number of benefits are associated with exoskeleton use, there are also factors to consider prior to using the device in therapy or for the long term.
Risks of using an exoskeleton
Robotic exoskeletons are generally safe when used with discretion. However, there are some risks associated with its use. Mild adverse effects that have been reported in research include: skin redness, small abrasions (i.e., scrapes), mild joint swelling, and mild bruising. Additionally, like other simple orthotics and braces, falls and fractures have been identified as a risk. It is suggested (weak evidence) that family and friends of exoskeleton users should be trained to deal with emergency situations, such as falls or the exoskeleton shutting off unexpectedly.
Considerations of using an exoskeleton in the community
While exoskeleton home use may seem promising, there are some limitations. These include:
- Slow walking speeds, which may not be ideal for everyday activities.
- High costs to purchase the device.
- Lack of availability of community-based exoskeletons.
- Limited capacity or poor efficiency in moving on uneven surfaces (e.g., hills, steps) or complex movements (turning, side-stepping, backwards walking).
- Being prone to water damage (they are not waterproof).
There are certain situations where extra attention is needed to determine whether robotic exoskeletons are appropriate and safe. Consult a qualified health provider for further safety information. Robotic exoskeletons are not recommended for individuals:
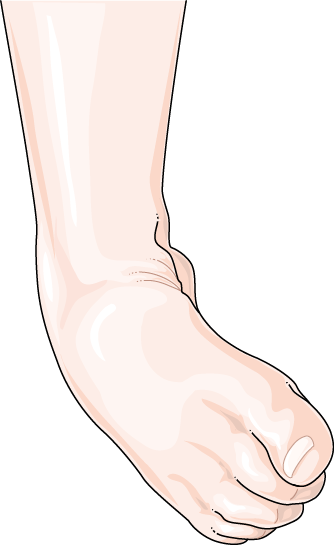
Extreme contractures are a contraindication for using exoskeletons.
- Who are unable to tolerate standing, even with an assistive device (walking frame, bracing), due to pain or other complications (autonomic dysreflexia, orthostatic hypotension).
- With severe neurological injuries (apart from SCI).
- With severe or uncontrolled spasticity.
- With osteoporosis.
- With fractures.
- With severe contractures (deformities that cause joint and muscle stiffness and limit normal or functional movement of the limbs).
Using Functional Electrical Stimulation (FES) to counter spasticityResearchers built a novel device that integrates FES into an exoskeleton to address the issue of severe spasticity affecting exoskeleton use. In this FES-exoskeleton hybrid, FES complemented the exoskeleton by stimulating tight extensor muscles to facilitate walking. The authors found that spasticity was temporarily reduced when FES was used when walking with an exoskeleton. Furthermore, it was found that the knee was more easily extended when moving from a sit-to-stand position, and the forces applied on the knee during sit-to-stand were reduced. While this (weak evidence) study provides some promise for individuals with severe spasticity to also use exoskeletons, further research is required. |
Using an exoskeleton requires practice. Although walking in an exoskeleton may seem daunting at first, research suggests that walking proficiency improves over time. In both newly injured (i.e., less than 6 months since injury) and chronically injured individuals with SCI, weak evidence shows that walking in the exoskeleton improves over time.
You get faster
Among newly injured individuals, weak evidence from one study suggests that walking speed in an exoskeleton becomes 3.2x faster after 25 1-hour training sessions. Moreover, these individuals were able to walk further in an exoskeleton after their training sessions. Among chronically injured individuals, similar trends are seen with increases in exoskeleton walking speed in two weak evidence studies. In another weak evidence study, it was found that 21 sessions were required to achieve the near-maximal walking speed at the end of a 12-week period, while 62 sessions were required to achieve near-maximal walking distance.
Less effort is Required
There is both weak and moderate evidence suggesting that the amount of effort it takes to walk using an exoskeleton decreases over time. This has been evaluated both subjectively (i.e., people feel like walking in an exoskeleton is not as hard over time) and physiologically (i.e., less demand on your body). This suggests that individuals are able to walk longer distances with lower effort after training to use an exoskeleton.
Is using an exoskeleton different for those with acute injuries versus chronic injuries?Researchers have noted differences in exoskeleton use among newly injured (e.g., in patients) and chronically injured individuals with respect to adverse effects and benefits received. Among people who have recently sustained an SCI, weak evidence indicates that the most common adverse effect was orthostatic hypotension (a sudden drop in blood pressure). One study found that orthostatic hypotension commonly occurred after the first stand or after pauses (e.g., to take vitals, or to turn). However, the frequency of orthostatic hypotension episodes tapered off after a couple of sessions. Furthermore, another study (weak evidence) suggests that newly injured individuals may see improvements in their independence and quality of life, whereas those with chronic injuries do not. More research is required to determine the significance of differences between acutely injured and chronically injured individuals who use exoskeletons. |
What factors influence walking speed?
Among individuals with SCI who use current exoskeleton devices, the average walking speed is 0.26 meters per second. This speed is fairly slow, and is lower than the average speed required to walk proficiently in the community (0.8 meters per second) and to cross the street safely (1.06 meters per second). However, walking speed in an exoskeleton is subject to improvement, depending on various factors.
Some factors that influence walking speed include age, level and type of injury, and the amount of training one receives. There is some (weak) evidence suggesting that those with incomplete, lower level SCI are more likely to exhibit faster walking speeds. In particular, one (weak evidence) study found that those with lower level paraplegia (i.e., T9-L1) were able to walk at significantly higher speeds. Moreover, there was a weak correlation between older age and faster walking speeds (i.e., older adults walk slightly faster than younger adults), though this could be related to the age-related difference in injury severity (that is, older individuals had lower levels of injury). No correlation was found with a greater time since injury.
What factors influence skill acquisition?
The time it takes to acquire proficient skills to walk in an exoskeleton varies greatly, ranging from 6-23 sessions. A variety of factors influence skill acquisition, including lifestyle, age, age at injury, and body mass index (BMI). Weak evidence suggests that an active lifestyle is the most important predictor of skill performance, although being younger and having a lower BMI are also associated with higher skill level. Additionally, the authors note that while having a lower level of injury was a positive predictor of skill between 2-4 weeks of using an exoskeleton, it did not predict final skill levels.
The type of exoskeleton being used may also influence skill acquisition. For example, weak evidence notes that a device with more support to the torso may facilitate skill acquisition as it provides more stability. Although we have summarized the research on factors influencing skill acquisition, the type of exoskeleton used in each study was not accounted for. As a result, we are unable to tease apart the effects of the aforementioned factors (e.g., lifestyle, age, BMI) and of the exoskeleton type on acquiring skills. The time it takes to acquire proficient skills to walk in an exoskeleton varies greatly, ranging from 6-23 sessions. A variety of factors influence skill acquisition, including lifestyle, age, age at injury, and body mass index (BMI). Weak evidence suggests that an active lifestyle is the most important predictor of skill performance, although being younger and having a lower BMI are also associated with higher skill level. Additionally, the authors note that while having a lower level of injury was a positive predictor of skill between 2-4 weeks of using an exoskeleton, it did not predict final skill levels.
Although walking in an exoskeleton is used primarily for rehabilitation purposes, the effort required to use the device is strenuous enough to be considered exercise. For example, one (weak evidence) study found that walking in an exoskeleton requires 3.34 times more effort than pushing a wheelchair, and 1.9 times more effort compared to walking without impairment, despite walking 7.4 times slower. Not surprisingly, participants also perceive themselves to be working harder. Participants from three (weak evidence) studies exercised at a moderate intensity, which is enough to get cardiovascular benefits, while walking in a robotic exoskeleton. Although some participants from a (weak evidence) study reported working at a low intensity, the authors noted that based on their heart rate and oxygen consumption, they were actually working at a moderate intensity. This suggests that some people may actually be working their bodies harder than they feel they are!
So why is walking in an exoskeleton so much work? Research suggests that using an exoskeleton requires a lot of work from the arms and torso to support an upright posture and to shift weight to initiate stepping. However, relative to other walking orthotics (e.g., robotic gait orthoses, hip-knee-ankle-foot orthoses), FES, and bracing, walking in an exoskeleton is considerably less effort. So then why would you use an exoskeleton for exercise if using other walking orthotics is harder work? The important consideration is stamina. You might work harder walking with rigid braces, but you may tire out quickly. With an exoskeleton, the understanding is that the assisted walking could allow you to exercise at moderate intensity for much longer.
Currently, there are only two exoskeleton models that have been approved for community use in North America. As such, there is limited evidence for using an exoskeleton in the community. In order to use an exoskeleton independently, individuals should be able to put on and take off the exoskeleton without professional help. Weak evidence has shown that individuals with paraplegia are able to independently put on and take off these devices, although those with tetraplegia are not. Additionally, weak evidence has shown that the time it takes to put on and take off an exoskeleton can be reduced with practice. Regarding walking speed in indoor versus outdoor environments, one weak evidence study has found that there are no significant differences in speed.
Some studies have looked at home- and community-based skills that can be completed using an exoskeleton. One weak evidence study found that the majority of participants could walk independently without a trainer in an exoskeleton, and perform tasks such as reaching high cupboards, using a stove, and using a sink, but were not able to walk on carpet and ramps. However, the authors note that some tasks were more difficult to complete in an exoskeleton, including reaching low cupboards and opening a fridge and getting items. Other (weak evidence) studies have found that a small proportion of people were able to do more advanced community-based tasks, such as entering/exiting elevators, operating automatic doors, navigating revolving doors, and ordering at a café. More research is required to determine how quickly individuals can pick up these skills.
There are currently many models of robotic exoskeletons continuing to be developed and refined. Exoskeletons are primarily used for rehabilitation purposes, although some models are available for community use. Using an exoskeleton has been shown to be relatively safe and easy to learn. Many benefits have been reported, including being able to ambulate, improvements in bone health, heart health, spasticity, bowel functioning, fitness, and pressure sores. While there are a lot of positive findings for robotic exoskeletons, this is an emerging field and stronger research is required to support these beneficial claims. A strong consideration is to weigh these benefits against cost, and also compare how these benefits compare to other to achieve similar gains.
For a review of how we assess evidence at SCIRE Community and advice on making decisions, please see SCIRE Community Evidence.
Parts of this page have been adapted from the SCIRE Project (Professional) “Lower Limb” Chapter:
Lam T, Wolfe DL, Domingo A, Eng JJ, Sproule S (2014). Lower Limb Rehabilitation Following Spinal Cord Injury. In: Eng JJ, Teasell RW, Miller WC, Wolfe DL, Townson AF, Hsieh JTC, Connolly SJ, Noonan VK, Loh E, McIntyre A, editors. Spinal Cord Injury Rehabilitation Evidence. Version 5.0. Vancouver: p 1-74.
Available from: scireproject.com/evidence/rehabilitation-evidence/lower-limb/
Evidence for “What are robotic exoskeletons?” is based on the following studies:
Khan, A. S., Livingstone, D. C., Hurd, C. L., Duchcherer, J., Misiaszek, J. E., Gorassini, M. A., … Yang, J. F. (2019). Retraining walking over ground in a powered exoskeleton after spinal cord injury: a prospective cohort study to examine functional gains and neuroplasticity, 1–17. Retrieved from https://doi.org/10.1186/s12984-019-0585-x
Ali, H. (2014). Bionic Exoskeleton: History, Development and the Future. IOSR Journal of Mechanical and Civil Engineering (IOSR-JMCE), 2014, 58–62. Retrieved from http://iosrjournals.org/iosr-jmce/papers/ICAET-2014/me/volume-5/12.pdf?id=7622
Gardner, A. D., Potgieter, J., & Noble, F. K. (2017). A review of commercially available exoskeletons’ capabilities. 2017 24th International Conference on Mechatronics and Machine Vision in Practice, M2VIP 2017, 2017–Decem, 1–5.
He, Y., Eguren, D., Luu, T. P., & Contreras-Vidal, J. L. (2017). Risk management and regulations for lower limb medical exoskeletons: A review. Medical Devices: Evidence and Research, 10, 89–107.
Evidence for “What is the history behind robotic exoskeletons?” is based on the following studies:
Yang, C. J., Zhang, J. F., Chen, Y., Dong, Y. M., & Zhang, Y. (2008). A review of exoskeleton-type systems and their key technologies. Proceedings of the Institution of Mechanical Engineers, Part C: Journal of Mechanical Engineering Science, 222(8), 1599–1612.
Ali, H. (2014). Bionic Exoskeleton: History, Development and the Future. IOSR Journal of Mechanical and Civil Engineering (IOSR-JMCE), 2014, 58–62. Retrieved from http://iosrjournals.org/iosr-jmce/papers/ICAET-2014/me/volume-5/12.pdf?id=7622
Evidence for “What are exoskeletons used for?” is based on the following studies:
Esquenazi, A., Talaty, M., Packel, A., & Saulino, M. (2012). The Rewalk powered exoskeleton to restore ambulatory function to individuals with thoracic-level motor-complete spinal cord injury. American Journal of Physical Medicine and Rehabilitation, 91(11), 911–921.
Kolakowsky-Hayner, S. A. (2013). Safety and Feasibility of using the EksoTM Bionic Exoskeleton to Aid Ambulation after Spinal Cord Injury. Journal of Spine.
Kozlowski, A. J., Bryce, T. N., & Dijkers, M. P. (2015). Time and effort required by persons with spinal cord injury to learn to use a powered exoskeleton for assisted walking. Topics in Spinal Cord Injury Rehabilitation, 21(2), 110–121.
Kressler, J., Thomas, C. K., Field-Fote, E. C., Sanchez, J., Widerström-Noga, E., Cilien, D. C., … Nash, M. S. (2014). Understanding therapeutic benefits of overground bionic ambulation: Exploratory case series in persons with chronic, complete spinal cord injury. Archives of Physical Medicine and Rehabilitation, 95(10), 1878-1887.e4.
Zeilig, G., Weingarden, H., Zwecker, M., Dudkiewicz, I., Bloch, A., & Esquenazi, A. (2012). Safety and tolerance of the ReWalkTM exoskeleton suit for ambulation by people with complete spinal cord injury: A pilot study. Journal of Spinal Cord Medicine, 35(2), 96–101.
Juszczak, M., Gallo, E., & Bushnik, T. (2018). Examining the effects of a powered exoskeleton on quality of life and secondary impairments in people living with spinal cord injury. Topics in Spinal Cord Injury Rehabilitation, 24(4), 336–342.
Khan, A. S., Livingstone, D. C., Hurd, C. L., Duchcherer, J., Misiaszek, J. E., Gorassini, M. A., … Yang, J. F. (2019). Retraining walking over ground in a powered exoskeleton after spinal cord injury: a prospective cohort study to examine functional gains and neuroplasticity, 1–17. Retrieved from https://doi.org/10.1186/s12984-019-0585-x
Karelis, A. D., Carvalho, L. P., Castillo, M. J. E., Gagnon, D. H., & Aubertin-Leheudre, M. (2017). Effect on body composition and bone mineral density of walking with a robotic exoskeleton in adults with chronic spinal cord injury. Journal of Rehabilitation Medicine, 49(1), 84–87.
Escalona, M. J., Brosseau, R., Vermette, M., Comtois, A. S., Duclos, C., Aubertin-Leheudre, M., & Gagnon, D. H. (2018). Cardiorespiratory demand and rate of perceived exertion during overground walking with a robotic exoskeleton in long-term manual wheelchair users with chronic spinal cord injury: A cross-sectional study. Annals of Physical and Rehabilitation Medicine, 61(4), 215–223.
Mcintosh, K., Charbonneau, R., Bensaada, Y., Bhatiya, U., & Ho, C. (2019). The Safety and Feasibility of Exoskeletal-Assisted Walking in Acute Rehabilitation After Spinal Cord Injury. Archives of Physical Medicine and Rehabilitation. Retrieved from https://doi.org/10.1016/j.apmr.2019.09.005
Stampacchia, G., Rustici, A., Bigazzi, S., Gerini, A., Tombini, T., & Mazzoleni, S. (2016). Walking with a powered robotic exoskeleton: Subjective experience, spasticity and pain in spinal cord injured persons. NeuroRehabilitation, 39(2), 277–283.
Baunsgaard, C. B., Nissen, U. V., Brust, A. K., Frotzler, A., Ribeill, C., Kalke, Y. B., … Benito Penalva, J. (2018). Exoskeleton gait training after spinal cord injury: An exploratory study on secondary health conditions. Journal of Rehabilitation Medicine, 50(9), 806–813.
Evidence for “What are the risks and considerations for using an exoskeleton?” is based on the following studies:
Tefertiller, C., Hays, K., Jones, J., Jayaraman, A., Hartigan, C., Bushnik, T., & Forrest, G. F. (2018). Initial outcomes from a multicenter study utilizing the indego powered exoskeleton in spinal cord injury. Topics in Spinal Cord Injury Rehabilitation, 24(1), 78–85.
Mcintosh, K., Charbonneau, R., Bensaada, Y., Bhatiya, U., & Ho, C. (2019). The Safety and Feasibility of Exoskeletal-Assisted Walking in Acute Rehabilitation After Spinal Cord Injury. Archives of Physical Medicine and Rehabilitation. Retrieved from https://doi.org/10.1016/j.apmr.2019.09.005
Mekki, M., Delgado, A. D., Fry, A., Putrino, D., & Huang, V. (2018). Robotic Rehabilitation and Spinal Cord Injury: a Narrative Review. Neurotherapeutics, 15(3), 604–617.
Miller, L. E., Zimmermann, A. K., & Herbert, W. G. (2016). [Miller, 2016] Clinical effectiveness and safety of powered exoskeleton-assisted walking on SCI patients, 455–466.
Van Herpen, F. H. M., Van Dijsseldonk, • R B, Rijken, • H, Keijsers, • N L W, Louwerens, J. W. K., & Van Nes, • I J W. (2019). Spinal Cord Series and Cases Case Report: Description of two fractures during the use of a powered exoskeleton. Retrieved from https://doi.org/10.1038/s41394-019-0244-2
Kandilakis, C., & Sasso-Lance, E. (n.d.). Exoskeletons for Personal Use After Spinal Cord Injury. Retrieved from https://doi.org/10.1016/j.apmr.2019.05.028
Evidence for “Are there restrictions or precautions for using robotic exoskeletons?” is based on the following studies:
Miller, L. E., Zimmermann, A. K., & Herbert, W. G. (2016). [Miller, 2016] Clinical effectiveness and safety of powered exoskeleton-assisted walking on SCI patients, 455–466.
Murray, S. A., Farris, R. J., Golfarb, M., Hartigan, C., Kandilakis, C., & Truex, D. (2018). FES Coupled with A Powered Exoskeleton for Cooperative Muscle Contribution in Persons with Paraplegia. Proceedings of the Annual International Conference of the IEEE Engineering in Medicine and Biology Society, EMBS, 2018–July, 2788–2792.
Ekelem, A., & Goldfarb, M. (2018). Supplemental stimulation improves swing phase kinematics during exoskeleton assisted gait of SCI subjects with severe muscle spasticity. Frontiers in Neuroscience, 12(JUN).
Evidence for “How does walking change over time?” is based on the following studies:
Mcintosh, K., Charbonneau, R., Bensaada, Y., Bhatiya, U., & Ho, C. (2019). The Safety and Feasibility of Exoskeletal-Assisted Walking in Acute Rehabilitation After Spinal Cord Injury. Archives of Physical Medicine and Rehabilitation. Retrieved from https://doi.org/10.1016/j.apmr.2019.09.005
Ramanujam, A., Momeni, K., Husain, S. R., Augustine, J., Garbarini, E., Barrance, P., … Forrest, G. F. (2018). Mechanisms for improving walking speed after longitudinal powered robotic exoskeleton training for individuals with spinal cord injury. Proceedings of the Annual International Conference of the IEEE Engineering in Medicine and Biology Society, EMBS, 2018–July, 2805–2808.
Tefertiller, C., Hays, K., Jones, J., Jayaraman, A., Hartigan, C., Bushnik, T., & Forrest, G. F. (2018). Initial outcomes from a multicenter study utilizing the indego powered exoskeleton in spinal cord injury. Topics in Spinal Cord Injury Rehabilitation, 24(1), 78–85.
Khan, A. S., Livingstone, D. C., Hurd, C. L., Duchcherer, J., Misiaszek, J. E., Gorassini, M. A., … Yang, J. F. (2019). Retraining walking over ground in a powered exoskeleton after spinal cord injury: a prospective cohort study to examine functional gains and neuroplasticity, 1–17. Retrieved from https://doi.org/10.1186/s12984-019-0585-x
Escalona, M. J., Brosseau, R., Vermette, M., Comtois, A. S., Duclos, C., Aubertin-Leheudre, M., & Gagnon, D. H. (2018). Cardiorespiratory demand and rate of perceived exertion during overground walking with a robotic exoskeleton in long-term manual wheelchair users with chronic spinal cord injury: A cross-sectional study. Annals of Physical and Rehabilitation Medicine, 61(4), 215–223.
Delgado, A. D., Escalon, M. X., Bryce, T. N., Weinrauch, W., Suarez, S. J., & Kozlowski, A. J. (2019). Safety and feasibility of exoskeleton-assisted walking during acute/sub-acute SCI in an inpatient rehabilitation facility: A single-group preliminary study. Journal of Spinal Cord Medicine. Retrieved from https://www.tandfonline.com/action/journalInformation?journalCode=yscm20
Baunsgaard, C. B., Nissen, U. V., Brust, A. K., Frotzler, A., Ribeill, C., Kalke, Y. B., … Benito Penalva, J. (2018). Exoskeleton gait training after spinal cord injury: An exploratory study on secondary health conditions. Journal of Rehabilitation Medicine, 50(9), 806–813.
Evidence for “What influences walking speed and skill acquisition when using an exoskeleton?” is based on the following studies:
Louie, D. R., Eng, J. J., & Lam, T. (2015). Gait speed using powered robotic exoskeletons after spinal cord injury: A systematic review and correlational study. Journal of NeuroEngineering and Rehabilitation, 12(1), 1–10. Retrieved from http://dx.doi.org/10.1186/s12984-015-0074-9
Hartigan, C., Kandilakis, C., Dalley, S., Clausen, M., Wilson, E., Morrison, S., … Farris, R. (2015). Mobility outcomes following five training sessions with a powered exoskeleton. Topics in Spinal Cord Injury Rehabilitation, 21(2), 93–99.
van Dijsseldonk, R. B., Rijken, H., W van Nes, I. J., van de Meent, H., W Keijsers, N. L., & W Keijsers, el L. (2019). Predictors of exoskeleton motor learning in spinal cord injured patients. Disability and Rehabilitation, 1-7.
Khan, A. S., Livingstone, D. C., Hurd, C. L., Duchcherer, J., Misiaszek, J. E., Gorassini, M. A., … Yang, J. F. (2019). Retraining walking over ground in a powered exoskeleton after spinal cord injury: a prospective cohort study to examine functional gains and neuroplasticity, 1–17. Retrieved from https://doi.org/10.1186/s12984-019-0585-x
Evidence for “Can I get exercise benefits from walking in an exoskeleton?” is based on the following studies:
Khan, A. S., Livingstone, D. C., Hurd, C. L., Duchcherer, J., Misiaszek, J. E., Gorassini, M. A., … Yang, J. F. (2019). Retraining walking over ground in a powered exoskeleton after spinal cord injury: a prospective cohort study to examine functional gains and neuroplasticity, 1–17. Retrieved from https://doi.org/10.1186/s12984-019-0585-x
Escalona, M. J., Brosseau, R., Vermette, M., Comtois, A. S., Duclos, C., Aubertin-Leheudre, M., & Gagnon, D. H. (2018). Cardiorespiratory demand and rate of perceived exertion during overground walking with a robotic exoskeleton in long-term manual wheelchair users with chronic spinal cord injury: A cross-sectional study. Annals of Physical and Rehabilitation Medicine, 61(4), 215–223.
Kozlowski, A. J., Bryce, T. N., & Dijkers, M. P. (2015). Time and effort required by persons with spinal cord injury to learn to use a powered exoskeleton for assisted walking. Topics in Spinal Cord Injury Rehabilitation, 21(2), 110–121.
Mcintosh, K., Charbonneau, R., Bensaada, Y., Bhatiya, U., & Ho, C. (2019). The Safety and Feasibility of Exoskeletal-Assisted Walking in Acute Rehabilitation After Spinal Cord Injury. Archives of Physical Medicine and Rehabilitation. Retrieved from https://doi.org/10.1016/j.apmr.2019.09.005
Evidence for “What evidence is there for using an exoskeleton in the community?” is based on the following studies:
Tefertiller, C., Hays, K., Jones, J., Jayaraman, A., Hartigan, C., Bushnik, T., & Forrest, G. F. (2018). Initial outcomes from a multicenter study utilizing the indego powered exoskeleton in spinal cord injury. Topics in Spinal Cord Injury Rehabilitation, 24(1), 78–85.
Hartigan, C., Kandilakis, C., Dalley, S., Clausen, M., Wilson, E., Morrison, S., … Farris, R. (2015). Mobility outcomes following five training sessions with a powered exoskeleton. Topics in Spinal Cord Injury Rehabilitation, 21(2), 93–99.
Khan, A. S., Livingstone, D. C., Hurd, C. L., Duchcherer, J., Misiaszek, J. E., Gorassini, M. A., … Yang, J. F. (2019). Retraining walking over ground in a powered exoskeleton after spinal cord injury: a prospective cohort study to examine functional gains and neuroplasticity, 1–17. Retrieved from https://doi.org/10.1186/s12984-019-0585-x
Spungen, A. M., Asselin, P. K., Fineberg, D. B., Kornfeld, S. D., & Harel, N. Y. (2012). Exoskeletal-Assisted Walking for Persons with Motor-Complete Paraplegia. VA Rehabilitation Research and Development National Center of Excellence for the Medical Consequences of Spinal Cord Injury. Retreived from: http://www.ryzur.com.cn/uploadfile/2016/0830/20160830115519272.pdf
Miller, L. E., Zimmermann, A. K., & Herbert, W. G. (2016). Clinical effectiveness and safety of powered exoskeleton-assisted walking on SCI patients. Medical Devices: Evidence and Research, 9:455–466.
Image credits
- Walking with a Clinician ©The SCIRE Community Team
- Hardiman I ©Bruce R. Fick and John B. Makinson, General Elerctric Co., Public Domain
- Active Suit ©Robotics Laboratory, Mihailo Pupin Institute
- Ekso Exoskeleton ©Ekso Bionics 2020
- ReWalk Exoskeleton ©ReWalk Robotics 2020
- REX Exoskeleton ©REX Bionics Ltd 2020
- Indego Exoskeleton ©Parker Hannifin Corp 2020
- ReWalk Exoskeleton ©ReWalk Robotics 2020
- Spasticity ©The SCIRE Community Team
- Colon ©Servier Medical Art, CC BY 3.0
- Femur ©Servier Medical Art, CC BY 3.0
- Modified from: Beating heart ©Lillit Kalachyan, CC BY 3.0
- Lightning ©FLPLF, CC BY 3.0
- ModifiedfromSpasticity ©The SCIRE Community Team
- Ankle sprain ©Servier Medical Art, CC BY 3.0
- Exoskeleton Icon ©The SCIRE Community Team
- Downtown New York City streets ©Free-photos, Pixabay License
- Candles ©The SCIRE Community Team
- Modified from: Weight Scale ©Sandra, CC BY 3.0
- Wheelchair Tennis ©Gan Khoon Lay, CC BY 3.0
- Personal user photo courtesy of Parker Hannifin Corporation, USA